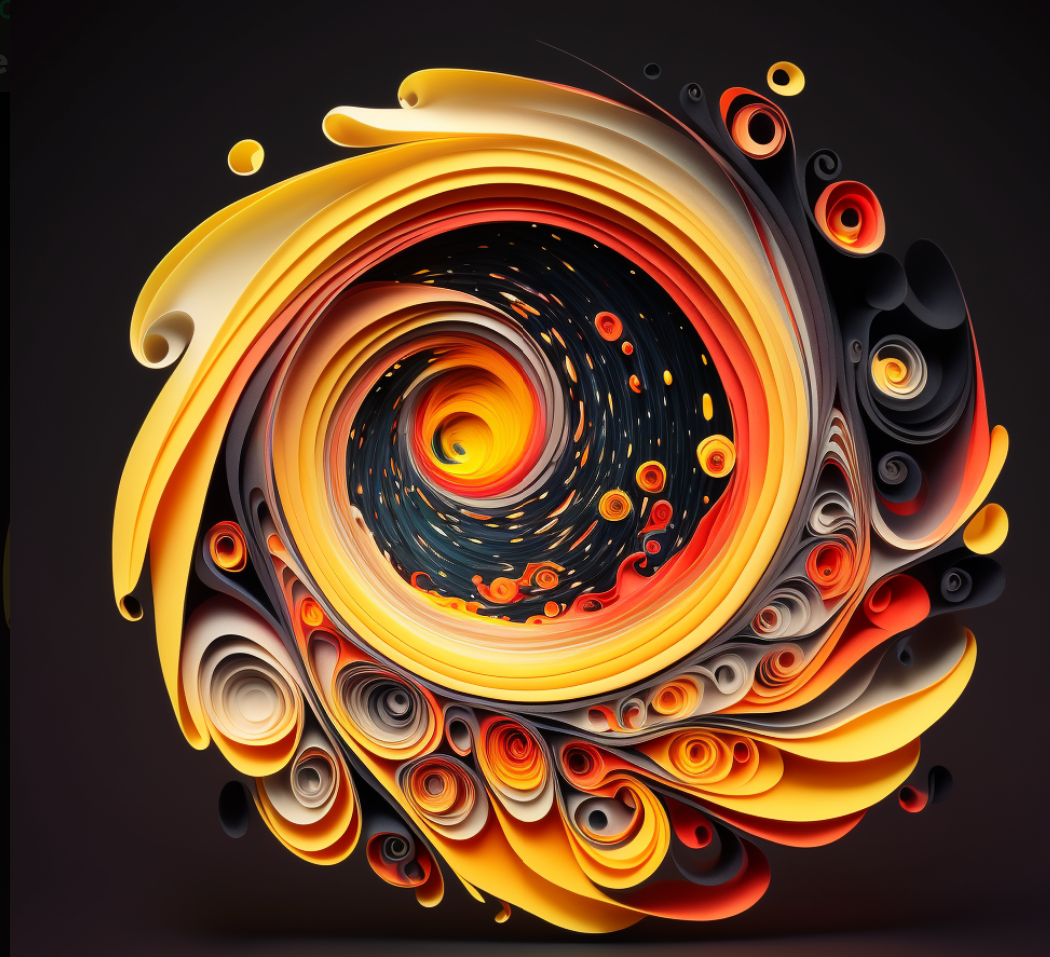
Chapter 3: The Solar System
Contents
1. Solar Nebula
-
A cloud of gas a dust begins to collapse under it’s own gravity causing it to flatten into a spinning disk known as the solar nebula. The density of particles in space reached a point that tipped the scale past equilibrium, This triggered the runaway gravitational collapse of matter in the cloud. As the cloud contracted into a disc, conservation of angular momentum caused the system to spin.
-
As the solar nebula continues to collapse, it heats up, and the pressure at its center becomes intense enough to initiate nuclear fusion, creating a new star, our Sun.
-
The solar nebula consists of gas and dust particles that begin to clump together due to gravitational attraction. These particles collided, sticking together to form meteors from new new minerals forming the first rocks and even larger bodies known as planetesimals. These planetesimals continued to grow through accretion, eventually becoming protoplanets.
-
The protoplanets continued to accrete material from the solar nebula until they became large enough to clear their orbits of debris, becoming fully formed planets.
It's tough to say when our solar system began. At one point, our area of space was full of gas and dust, and it got so dense that it compressed everything into a flat, spinning disc.
It marks the beginning of the Chaotian Eon.
2. Chaotian Eon
The stages of our solar system formation happened before the start of geologic time, because earth has not formed yet. Geologic Time is a naming and dating classification system based on events on earth, we will explore a small part of the geologic time scale each chapter.
The nomenclature for geologic time periods prior to the formation of Earth remains a topic of debate among scientists. One proposed timeline begins with the Chaotian Eon marked by the accretion of the solar nebula. This Eon is subdivided into two eras representing the time before the sun reached the point of generating sunlight from fusion. The first era is called the Eochaotian. Once the light and electromagnetic energy from the sun filled the solar system the second era began, called the Neochaotian.
-
The Chaotian Eon starts with the accretion of the solar nebula and ends with the impact of Theia with another protoplanet to form The Earth and moon.
-
The Eochaotian Era, begins when the Solar Nebula began to condense under it’s own gravity. It includes the Nephelean Period, for the cloud that is the nebula, and the Erebrean Period, for the proto-Sun that was not yet luminous.
-
The second era, Neochaotian, starts with the first light from the Sun and comprises the Hyperitian Period, named after the Titan Sun god Hyperion, for the time when the Sun's first light was brighter than its subsequent main sequence, and the Titanomachean Period, named after the war of the Titans, to encompass the collision of proto-planets that formed our present set of planets.
3. Asteroids, Meteors, & Meteorites
An asteroid is a small rocky object that orbits the sun, a meteor is typically a smaller part of an asteroid which has entered the earth’s atmosphere, if it survives the burnup and lands on the ground, the remaining debris are considered meteorites. Since the earth hasn’t formed yet, we will considerer the rocks flying around the young solar system asteroids.
Asteroids were abundant in the young solar system, their collision led to the formation of protoplanets. Remnants of ancient asteroid impacts along with analysis of modern meteorite samples allow us to study the geologic processes that occurred during the early stages of the solar system’s formation.
Meteorites found on Earth are classified by their chemical composition with two primary endmembers: quartz rich or stony meteorites and iron rich meteorites.
-
Stony meteorites are the most common meteorite, accounting for 80% of all known meteorites.
Stony meteorites are thought to be among the oldest objects in the solar system and provide important insights into the formation and evolution of the planets. They are believed to have formed in the early solar system from the same dust and gas that eventually gave rise to the planets.
Stony meteorites are typically classified into two groups: chondrites and achondrites. Chondrites are characterized by the presence of chondrules, which are small, round mineral grains formed from the rapid cooling of molten droplets in the early solar system. Chondrites are further classified into several groups based on their chemical, mineralogical, and isotopic compositions, such as ordinary chondrites (H, L, and LL), carbonaceous chondrites (CI, CM, CO, CR, CV, and CK), enstatite chondrites (EH and EL), R chondrites, and K chondrites.
These subtypes provide valuable information about the early history of the solar system, such as the conditions in the solar nebula and the processes that led to the formation of the planets.
Stony meteorites are rich with silicate minerals such as olivine, pyroxene, and plagioclase feldspar.
Stony meteorites are often heavily cratered and show signs of weathering due to their long exposure to the Earth's atmosphere. They are highly prized by collectors and scientists alike for their unique insights into the formation and evolution of our solar system.
-
Iron meteorites are a type of meteorite that is composed primarily of iron and nickel, with small amounts of other elements such as cobalt, phosphorus, and carbon. They are relatively rare, accounting for only about 5-10% of all known meteorites.
Iron meteorites are believed to have originated from the cores of ancient asteroids or planetesimals that were destroyed by collisions in the early solar system. They are often heavily metallic and can have a distinctive crystalline structure known as a Widmanstätten pattern, which is formed by slow cooling over millions of years.
Iron meteorites are classified into several groups based on their mineral and chemical compositions, including hexahedrites, octahedrites, and ataxites. These groups are differentiated by the size and shape of the metallic crystals in the meteorite, as well as the amount of other elements present.
Iron meteorites are often recognized by their distinctive appearance, which is different from most rocks found on Earth. They are often heavily pitted and weathered due to their long exposure to the Earth's atmosphere. Iron meteorites are highly prized by collectors and scientists for their unique insights into the formation and evolution of our solar system, as well as their potential applications in technology and industry due to their high iron and nickel content.
-
-
Achondrites: Achondrites lack chondrules and have a more differentiated composition, indicative of igneous processes on their parent bodies. This group includes several subcategories, such as HED (howardite, eucrite, and diogenite) meteorites, lunar meteorites, Martian meteorites, and other rare achondrite types like angrites and ureilites.
-
Ordinary chondrites (H, L, and LL): These are the most abundant type of chondrites, accounting for about 86% of all chondrite meteorite falls. They are primarily composed of olivine and pyroxene, with lesser amounts of plagioclase feldspar, iron-nickel metal, and troilite (FeS). Ordinary chondrites are further subdivided into H (high iron), L (low iron), and LL (low iron, low metal) groups based on their metal content and other compositional differences.
-
Carbonaceous chondrites (CI, CM, CO, CR, CV, and CK): These chondrites are characterized by a higher abundance of volatile elements, such as water and organic compounds, compared to other chondrite groups. They contain a variety of minerals, including olivine, pyroxene, serpentine, and magnetite, as well as carbonaceous material, like insoluble organic matter, and hydrated minerals. Carbonaceous chondrites are important for studying the early solar system's chemistry, particularly the delivery of water and organic materials to Earth.
These meteorites are among the rarest in and most important because they represent material formed very early in the history of our solar system, before the formation of the planets. They are named for the tiny inclusions called chondrules which are debris from the early solar system. Less than 5 percent of these are considered carbonaceous, meaning they contain a relatively high content of carbon and often contain trace water. It’s thought they formed far away from the sun and delivered volatile material to the newly forming Earth. One of the largest and most studies is the Allende meteorite.
-
Enstatite chondrites (EH and EL): These chondrites are composed mainly of enstatite (an Mg-rich pyroxene) and contain almost no oxidized iron. They are further subdivided into EH (high iron) and EL (low iron) groups. Enstatite chondrites are notable for their high levels of the element iridium, which is rare in the Earth's crust.
-
Also known as R chondrites, Rumuruti chondrites are a rare group of meteorites named after their type specimen, the Rumuruti meteorite, which fell in Kenya in 1934. They are characterized by their unique chemical and mineralogical properties that distinguish them from other chondrite groups. R chondrites account for less than 1% of all known chondrites and are thought to have originated from a distinct parent body in the solar system.
Mineralogically, R chondrites are composed primarily of olivine and pyroxene, with lesser amounts of plagioclase, phosphates, Fe-Ni metal, and sulfides. Unlike other chondrite groups, R chondrites have a high abundance of oxidized iron, with very little metallic iron. This high degree of oxidation indicates that the parent body of R chondrites experienced a more oxidizing environment during its formation compared to the parent bodies of other chondrite groups.
Chemically, R chondrites show a distinct depletion in volatile elements, such as alkali and alkaline earth elements, compared to other chondrite groups. Their bulk composition is also characterized by relatively high concentrations of refractory elements like scandium, zirconium, and hafnium. These compositional differences suggest that R chondrites formed under unique conditions in the early solar system, possibly in a different region or from a different reservoir of materials compared to other chondrite groups.
Oxygen isotopic compositions of R chondrites are distinct as well, falling in a separate region on a three-isotope oxygen plot. This further supports the idea that R chondrites formed from a different reservoir of materials in the early solar system.
The exact formation and history of R chondrites are still not fully understood, and ongoing research seeks to uncover more information about their parent body and the processes that led to their unique characteristics. By studying R chondrites and other meteorite groups, scientists can learn more about the diverse conditions and processes that occurred in the early solar system.
-
K chondrites are an extremely rare group of meteorites, and their classification is not as well-established as other chondrite groups. The "K" in their name stands for Kakangari, named after the type specimen that fell in Tamil Nadu, India, in 1890. Due to their rarity and limited number of samples, the information about K chondrites is limited, and their characteristics are not as well-defined as other chondrite groups.
Mineralogically, K chondrites are composed mainly of olivine, pyroxene, and plagioclase, with minor amounts of Fe-Ni metal, sulfides, and other accessory minerals. They also contain chondrules, which are small, round mineral grains formed from the rapid cooling of molten droplets in the early solar system.
Chemically, K chondrites show an unusual composition that includes a higher proportion of alkali elements like sodium and potassium compared to other chondrite groups. The enrichment in alkali elements suggests that K chondrites might have formed in a different region of the early solar system or from a different reservoir of materials.
The oxygen isotopic composition of K chondrites is also distinct from other chondrite groups, supporting the idea that they have a unique origin. However, due to the limited number of samples and the rarity of K chondrites, their exact formation history and relationship to other meteorite groups are not yet fully understood.
Further research and analysis of K chondrites and other rare meteorite groups are necessary to gain a more comprehensive understanding of their origins, formation processes, and the diverse conditions that existed in the early solar system.
-
One type of chondrule called a “calcium-aluminum rich inclusion” (CAI) contain the rare isotope of magnesium-26 which forms from the decay of aluminum-26. This decay happens rapidly and happened soon after the formation of the solar system and indicates they formed due to a massive shockwave of a supernova that may have triggered the formation of the solar system. These meteorites contain exotic forms of calcium, barium and neodymium along with krypton, xenon, nitrogen and other rare elements.
-
-
The Widmanstätten texture, also known as the Widmanstätten pattern or structure, is a unique characteristic found in the interior of some iron meteorites and certain pallasite meteorites. It was named after Count Alois von Beckh Widmanstätten, an Austrian scientist who independently discovered the pattern in 1808, although it was first observed by English mineralogist William Thomson in 1804.
The Widmanstätten texture is a geometric pattern of intersecting bands or plates that results from the slow cooling of a Fe-Ni (iron-nickel) alloy over millions of years within the parent bodies of these meteorites. This slow cooling allows the two main metallic phases, kamacite (α-Fe,Ni) and taenite (γ-Fe,Ni), to separate and form an intergrowth of crystal structures.
Kamacite is a low-nickel phase (5-10% Ni) with a body-centered cubic structure, while taenite is a high-nickel phase (20-50% Ni) with a face-centered cubic structure. During the cooling process, the kamacite forms as elongated bands or plates, while the taenite remains as a matrix between the kamacite bands.
When a meteorite with the Widmanstätten texture is cut, polished, and etched with a mild acid, the pattern becomes visible. The kamacite bands are more susceptible to acid etching than the taenite, so the pattern appears as a series of light and dark bands forming angular or cross-like structures.
The presence of the Widmanstätten texture is diagnostic of meteoritic origin and is not found in terrestrial iron-nickel alloys. The texture can provide insights into the cooling rates and thermal history of the meteorite's parent body, as well as information about the formation and evolution of the early solar system.
-
Stony-Iron Meteorites - rare at less than 1% of meteorites, they are metallic & stony, equal parts iron (Fe) -nickel (Ni) metal and silicate minerals including gemstones. The two main types include:
Pallasites - a type of stony-iron meteorite containing large olivine (Mg2SiO4/Fe2SiO4) crystals embedded within a metal framework. These are thought to represent material within the mantle of Earth. Oldest example is the Fukang Meteorite > 4.5 Bya which is also one of the most beautiful with a honeycomb mosaic of olivine crystals.
Mesosiderites - a type of stony-iron meteorite containing broken fragments of rocks and minerals in a fine grained matrix including olivine (Mg2SiO4/Fe2SiO4), pyroxene (Ca, Na, Fe II, Mg and Cr, Al, Mg, Co Mn with Si, Al2O6) and Ca Feldspar (CaAl2Si2O8/NaAlSi3O8). These form from the debris after a collision of asteroids, molten metal mixes with solid fragments of silicate rocks.
-
-
Pallasites are a rare and fascinating group of stony-iron meteorites, which constitute approximately 1-2% of all known meteorites. They are named after the German naturalist Peter Simon Pallas, who first described the Krasnojarsk pallasite found in Siberia in 1772. Pallasites are of particular interest to scientists due to their unique composition and the insights they provide into the processes that occurred within the parent bodies of these meteorites.
Pallasites are characterized by a mixture of metallic and silicate components. They primarily consist of large, translucent to transparent olivine (a magnesium-iron silicate mineral) crystals embedded in a matrix of Fe-Ni (iron-nickel) metal, which is composed of kamacite and taenite. This stunning combination of greenish-yellow olivine crystals and shiny metallic regions makes pallasites visually striking and highly sought after by collectors.
The exact origin of pallasites is still a subject of debate among scientists. One of the leading theories suggests that pallasites formed at the core-mantle boundary of differentiated parent bodies, such as asteroids or protoplanets. In this scenario, the Fe-Ni metal represents the core material, and the olivine crystals represent the mantle material. Due to the heating and pressure conditions at the core-mantle boundary, these materials mixed and then solidified to form pallasites. Alternatively, some researchers propose that pallasites could have formed through impact processes, where the metallic core and silicate mantle materials of a differentiated body were mixed during a high-energy collision.
Pallasites are generally classified into two main groups: main-group pallasites (MGP) and Eagle Station pallasite grouplet (ESP)
-
Main Group Pallasites (MGP) are the most common and well-known type of pallasites, which are stony-iron meteorites composed of a mixture of metallic and silicate components. As mentioned earlier, pallasites are characterized by their striking appearance, featuring large, translucent to transparent olivine crystals embedded in a matrix of Fe-Ni (iron-nickel) metal composed of kamacite and taenite.
Composition and Structure: The olivine crystals in main group pallasites are predominantly magnesium-rich (forsterite), with minor amounts of iron. The Fe-Ni metal matrix is a combination of kamacite (low nickel content) and taenite (high nickel content), with minor amounts of other metallic phases and sulfides, such as troilite (FeS). The unique structure of main group pallasites, with the intergrowth of silicate and metallic phases, is believed to provide valuable information about the core-mantle boundary in their parent bodies.
Origin: The prevailing theory for the formation of main group pallasites suggests they originated at the core-mantle boundary of differentiated parent bodies, such as asteroids or protoplanets. In this scenario, the Fe-Ni metal represents the core material, while the olivine crystals represent the mantle material. These materials mixed due to the heating and pressure conditions at the core-mantle boundary and then solidified to form pallasites. However, alternative hypotheses propose that pallasites could have formed through impact processes, where metallic core and silicate mantle materials were mixed during a high-energy collision.
Oxygen Isotopes and Parent Bodies:
The oxygen isotopic composition of main group pallasites is relatively homogeneous and distinct from other meteorite groups. This homogeneity indicates that the MGPs likely originated from a single or a few closely related parent bodies. By studying the oxygen isotopes and other geochemical properties of main group pallasites, scientists can gain insights into the formation and evolution of their parent bodies and the early solar system.
Overall, main group pallasites provide a unique opportunity for scientists to study the processes that occurred within the parent bodies of these meteorites, offering insights into planetary formation, differentiation, and the early history of our solar system.
-
The Eagle Station Pallasite Grouplet (ESP) is a small and distinct group of pallasite meteorites that differ in composition and characteristics from the more common Main Group Pallasites (MGP). The ESP is named after the Eagle Station meteorite, which was found in Kentucky, USA, in 1950. The grouplet consists of only a few known meteorites, making them quite rare and scientifically interesting.
Composition and Structure:
Like other pallasites, the Eagle Station Pallasite Grouplet is composed of a mixture of metallic and silicate components. ESPs contain large olivine crystals embedded in a matrix of Fe-Ni (iron-nickel) metal, which includes kamacite and taenite. However, the olivine crystals in ESPs are generally more iron-rich (fayalite) compared to the magnesium-rich (forsterite) olivine crystals found in MGPs. The Fe-Ni metal in ESPs also has a slightly different composition, with higher levels of certain trace elements such as gallium (Ga) and germanium (Ge).
Origin:
The exact origin of the Eagle Station Pallasite Grouplet, like other pallasites, is still a subject of debate among scientists. One leading hypothesis suggests that ESPs, similar to MGPs, formed at the core-mantle boundary of a differentiated parent body (e.g., asteroid or protoplanet). In this scenario, the Fe-Ni metal represents the core material, while the olivine crystals represent the mantle material. The distinct chemical composition of ESPs compared to MGPs could imply that they originated from a different parent body or experienced different processing conditions.
Another hypothesis proposes that pallasites, including ESPs, formed through impact processes, where the metallic core and silicate mantle materials of a differentiated body were mixed during a high-energy collision. This impact scenario could also explain the differences in composition between ESPs and MGPs.
Oxygen Isotopes and Parent Bodies:
The oxygen isotopic composition of the Eagle Station Pallasite Grouplet is distinct from that of the Main Group Pallasites, indicating that they likely originated from a separate or distinct parent body. Studying the oxygen isotopes and other geochemical properties of ESPs provides valuable insights into the formation and evolution of their parent bodies and the early solar system.
In summary, the Eagle Station Pallasite Grouplet represents a rare and distinct group of pallasite meteorites, offering unique insights into planetary formation, differentiation, and the processes that occurred within their parent bodies.
Explore The Meteoritical Society Database
5. ProtoPlanets and Planets
Protoplanets are built up from dust and gas particles in this process, they stick together through electrostatic forces, forming larger and larger bodies becoming planetesimals, which are large enough to begin accumulating gas from the surrounding disk. As these planetesimals grow, they begin to exert a gravitational pull on other objects in the disk, leading to more collisions and the formation of even larger objects.
Jupiter is thought to have formed first because it is the largest planet in our solar system and has a mass that is greater than all the other planets combined.
Jupiter's size and mass allowed it to gravitationally attract and accrete gas and dust more efficiently than the other planets in our solar system, which helped it to form faster than the rest. Additionally, its location in the solar system, further from the Sun than the inner planets, allowed it to accumulate more gas and dust over time, making it even larger.
-
The four inner planets - Mercury, Venus, Earth, and Mars - are called terrestrial planets because they are rocky and have a solid surface. These planets are made up mostly of metal and rock, with their cores being mostly composed of iron and nickel.
-
Mercury, the closest planet to the Sun, is believed to have formed through a process of core accretion, similar to the gas giants. However, because of its proximity to the Sun, the protoplanetary disk in which it formed was much hotter and less dense than those farther out in the solar system. This made it more difficult for the small particles of dust and gas to stick together and form larger planetesimals, resulting in the formation of a relatively small planet.
Mercury's composition is dominated by metal and rock, with a high density suggesting a large metallic core. In fact, it has the largest core of any planet in the solar system, making up approximately 85% of its total volume. The core is composed primarily of iron, with smaller amounts of nickel and other elements. Surrounding the core is a silicate mantle, which is much thinner than those of the other terrestrial planets.
Mercury's geologic history is characterized by a period of intense volcanic activity that created large plains and extensive lava flows. This was followed by a period of contraction as the planet cooled, resulting in the formation of scarps and wrinkles on the surface. The planet also experienced impacts from asteroids and comets, which created craters and basins
-
Venus's composition is similar to that of Earth, with a metal core surrounded by a mantle and crust composed primarily of silicates. However, it has a much thicker and denser atmosphere than Earth, composed mostly of carbon dioxide with trace amounts of nitrogen and other gases. This thick atmosphere traps heat from the Sun, leading to surface temperatures that are hotter than those on Mercury, despite Venus being farther away from the Sun. The extreme conditions on Venus make it difficult to study its surface directly, but data from spacecraft and radar imaging have revealed a geologically active planet with a complex surface.
Venus's geologic history is characterized by extensive volcanic activity that has resurfaced much of the planet. This activity has created vast plains and mountains, as well as features such as lava channels and volcanoes. The planet has also experienced tectonic activity, with evidence of large rifts and fault systems. The thick atmosphere has led to a lack of erosion and weathering, preserving many of these features for billions of years. While Venus is often referred to as Earth's "sister planet," its extreme conditions and unique geologic history make it a fascinating subject for study and comparison.
-
Earth is the third planet from the Sun and the largest of the terrestrial planets. It is believed to have formed about 4.5 billion years ago through a process of accretion, in which dust and gas particles in the early solar system came together to form planetesimals, which then collided and merged to form larger bodies.
Earth's composition is dominated by a metallic core composed primarily of iron, nickel, and other elements. Surrounding the core is a mantle composed of silicates, and on top of the mantle is the Earth's crust, which is divided into tectonic plates that move and interact with each other, creating earthquakes, volcanic eruptions, and mountain ranges.
Earth's geologic history is characterized by a long and complex series of processes, including plate tectonics, erosion, weathering, and volcanic activity. These processes have shaped the surface of the planet and created a diverse range of landforms, including mountains, valleys, canyons, and plains. The planet's atmosphere, which is composed mainly of nitrogen and oxygen, supports a wide variety of life, including complex organisms such as animals and plants.
-
Mars is the fourth planet from the Sun and is a rocky, terrestrial planet similar to Earth in many ways. It is believed to have formed through a process of accretion, in which dust and gas particles in the early solar system came together to form planetesimals, which then collided and merged to form larger bodies.
Mars's composition is dominated by a metallic core composed primarily of iron, with a surrounding mantle composed of silicates. The planet's thin atmosphere is composed mostly of carbon dioxide, with trace amounts of nitrogen and other gases. Mars has a relatively small size and a lower density than Earth, which has led to a weaker gravitational field and a more tenuous atmosphere.
Mars's geologic history is characterized by a period of intense volcanic activity that created many of the planet's largest features, including the largest volcano in the solar system, Olympus Mons. The planet also experienced impacts from asteroids and comets, which created many of the craters and basins that are visible on its surface today. Mars also has a history of water on its surface, with evidence of dried-up riverbeds and other features suggesting that liquid water once flowed on the planet's surface.
The planet's thin atmosphere and weak magnetic field have led to significant erosion and weathering of its surface, and its current conditions are much colder and drier than those on Earth. Despite these challenges, Mars remains a fascinating subject of study and exploration, with the potential to shed light on the processes of planetary formation and evolution, as well as the prospects for life beyond our own planet.
-
The asteroid belt is a region of the solar system located between the orbits of Mars and Jupiter, where many small, rocky objects known as asteroids or minor planets orbit the Sun. It is estimated to contain over a million objects, with sizes ranging from just a few meters to several hundred kilometers in diameter.
The formation of the asteroid belt is still a subject of active research and debate among scientists, but one widely accepted theory is that it was formed from the debris left over after the formation of the solar system. According to this theory, the asteroid belt is composed of fragments of rock and metal that never coalesced into a planet due to the strong gravitational pull of Jupiter, which prevented the material from coming together into a single body. This theory is supported by observations that suggest that the asteroids in the belt are composed of similar material to the terrestrial planets, such as Earth and Mars.
The asteroid belt is not a uniform, densely packed region, but instead consists of many individual objects in widely varied orbits. The asteroid belt is relatively stable, with the majority of the asteroids maintaining their current orbits for millions of years. However, collisions between asteroids can occur, and some asteroids may be perturbed into new orbits by the gravity of the gas giants or other objects in the solar system.
The study of asteroids in the asteroid belt is important for understanding the early solar system, as well as for gaining insight into the potential for planetary defense against asteroids that may pose a threat to Earth. In recent years, spacecraft missions such as NASA's Dawn and OSIRIS-REx have visited and studied asteroids in the asteroid belt, providing new insights into their composition, structure, and history.
-
The four outer planets - Jupiter, Saturn, Uranus, and Neptune - are called gas giants because they are composed mostly of gas and do not have a solid surface. These planets are mostly made up of hydrogen and helium, with small amounts of heavier elements such as oxygen, carbon, and nitrogen. These planets have cores too, but they are not solid like the terrestrial planets. Instead, they are made up of rock, metal, and ice, and are thought to be under such extreme pressure that they behave like liquids.
-
Jupiter is the largest planet in our solar system, with a diameter of over 86,000 miles (138,000 kilometers) - about 11 times that of Earth. It is a gas giant planet, composed mostly of hydrogen and helium, with traces of other elements such as methane, ammonia, and water vapor. Its size and mass are so great that it has an intense gravitational pull, which has shaped the orbits of other planets and objects in our solar system.
Jupiter's atmosphere is characterized by colorful cloud bands and a massive storm known as the Great Red Spot, which has been raging for at least 350 years. The planet also has dozens of moons, including four large moons known as the Galilean moons, which were discovered by Galileo Galilei in 1610. These moons are among the most interesting objects in the solar system, with evidence of subsurface oceans and the potential for harboring life.
Jupiter's formation is believed to have occurred through a process of core accretion, similar to the formation of other gas giants. However, its location in the solar system allowed it to accumulate much more gas and dust than the other planets, leading to its massive size. Jupiter has a complex internal structure, with a rocky core thought to be larger than Earth, surrounded by layers of liquid metallic hydrogen and helium. Understanding the formation and evolution of Jupiter is important for understanding the origins and characteristics of other gas giants, as well as the role of giant planets in the formation and evolution of planetary systems.
-
Saturn, like Jupiter, is a gas giant planet that is believed to have formed through a process of core accretion in the early solar system. This process involves the gradual accumulation of gas and dust particles in a protoplanetary disk, which eventually leads to the formation of planetesimals - the building blocks of planets.
As these planetesimals grow larger through collisions and accretion, they begin to exert a gravitational pull on other objects in the disk, leading to more collisions and the formation of even larger objects. Saturn's size and mass allowed it to accrete gas and dust more efficiently than the other planets in the solar system, which helped it to form faster than the rest. Its location in the solar system, further from the Sun than the inner planets, allowed it to accumulate more gas and dust over time, making it even larger.
Saturn's core is thought to be composed of rock, metals, and ice, surrounded by layers of liquid metallic hydrogen and helium. Its outer atmosphere is composed primarily of hydrogen and helium, with traces of other elements such as methane, ammonia, and water vapor. Saturn is also known for its impressive ring system, which is composed of countless particles of ice and rock that orbit the planet.
-
Uranus is the seventh planet from the Sun and is classified as an ice giant, a type of planet that is intermediate in size and composition between the gas giants and the terrestrial planets. Uranus is unique among the planets in our solar system because its axis of rotation is tilted at an extreme angle, causing its seasons to last for decades.
Uranus's composition is dominated by ices, such as water, methane, and ammonia, with a smaller rocky core thought to be about the size of Earth. The planet's atmosphere is composed mostly of hydrogen and helium, with traces of other gases such as methane, which gives Uranus its distinctive blue-green color.
The formation of Uranus is believed to have occurred through a process of core accretion, similar to the gas giants. However, its location in the solar system, farther from the Sun than Jupiter and Saturn, meant that there was less material available for it to accrete. This may have led to a slower and more prolonged formation process, with Uranus possibly forming from a mix of ices and rocky material.
Uranus has a complex system of rings and over two dozen moons, including some that are thought to be among the largest and most intriguing objects in the solar system. The planet's unique features, including its extreme axial tilt and icy composition, make it a fascinating subject of study and exploration, with the potential to shed light on the processes of planetary formation and evolution in our solar system and beyond.
-
Neptune is the eighth and farthest planet from the Sun in our solar system, classified as an ice giant planet like Uranus. It is a large and gaseous planet, similar in many ways to Uranus, with a diameter of about 30,000 miles (49,000 kilometers) - almost four times that of Earth.
Neptune's composition is dominated by ices, such as water, methane, and ammonia, along with a rocky core that is about the size of Earth. Its atmosphere is composed mostly of hydrogen and helium, with traces of methane that gives it a blue color similar to that of Uranus.
Like Uranus, Neptune is believed to have formed through a process of core accretion, in which dust and gas particles in the early solar system came together to form planetesimals, which then collided and merged to form larger bodies. Neptune's location in the solar system, far from the Sun, allowed it to accumulate more gas and dust over time, making it larger than Uranus.
Neptune has a complex system of rings and over a dozen moons, including Triton, which is one of the largest and most interesting objects in the solar system. Triton is thought to be a captured object from the Kuiper Belt, a region of the solar system beyond Neptune that is rich in icy and rocky debris.
-
Pluto is a dwarf planet located in the outer reaches of our solar system, in a region called the Kuiper Belt. It was discovered in 1930 by American astronomer Clyde Tombaugh and was classified as the ninth planet in the solar system until 2006, when it was reclassified as a dwarf planet.
Pluto is much smaller than the eight planets in the solar system and has a diameter of only about 1,400 miles (2,377 kilometers), which is less than one-fifth the diameter of Earth. It is also much less massive than the eight planets, making it less able to clear its orbit of debris and thus leading to its reclassification as a dwarf planet.
Pluto has a highly elliptical orbit that takes it from about 30 to 50 astronomical units (AU) from the Sun, with a period of 248 Earth years. It has five known moons, the largest of which, Charon, is almost half the size of Pluto itself.
Pluto's surface is composed mainly of ice and rock, with a thin atmosphere of nitrogen, methane, and carbon monoxide. The surface of Pluto is covered in craters, mountains, and vast plains of ice, with regions of different colors and composition that suggest a complex geologic history.
The exploration of Pluto has been limited to a few spacecraft flybys. In 2015, NASA's New Horizons mission made the first close-up observations of Pluto and its moons, revealing a world far more complex and diverse than previously imagined. The mission provided detailed images and measurements of Pluto's surface, atmosphere, and geology, shedding new light on the history and composition of this distant world.
-
The Kuiper Belt is a vast region of the solar system beyond the orbit of Neptune, extending from about 30 to 50 astronomical units (AU) from the Sun. It is named after Dutch-American astronomer Gerard Kuiper, who first predicted the existence of such a region in the early 1950s.
The Kuiper Belt is similar in many ways to the asteroid belt between Mars and Jupiter, but it is much larger and contains more objects. The belt is believed to be composed of countless small, icy objects, including dwarf planets such as Pluto and Eris, as well as a variety of smaller bodies, such as comets, asteroids, and Kuiper Belt objects (KBOs).
The Kuiper Belt is thought to be the source of many of the comets that periodically pass through the inner solar system. These comets are believed to originate from the outer reaches of the belt, where they are perturbed by the gravity of the gas giants and sent on orbits that bring them closer to the Sun. Some KBOs have highly eccentric orbits that take them far from the Sun, while others have more circular orbits that keep them closer to the Sun.
Bulk Composition of Earth
The bulk composition of Earth is dominated by a few key elements. Here are the most abundant elements in Earth's overall composition by mass:
Iron (Fe) - 34.6%
Oxygen (O) - 29.5%
Silicon (Si) - 15.2%
Magnesium (Mg) - 12.7%
Sulfur (S) - 2.4%
Nickel (Ni) - 1.9%
Calcium (Ca) - 1.5%
Aluminum (Al) - 1.4%
Other elements, such as sodium, potassium, and trace elements, make up less than 1% of Earth's total mass.
Bulk Composition of Earth’s Crust
The composition of the crust is different from the bulk composition of Earth because lighter elements have migrated to the surface over time. Here are the most abundant elements in Earth's crust by mass:
Oxygen (O) - 46.6%
Silicon (Si) - 27.7%
Aluminum (Al) - 8.1% SIGNIFICANT INCREASE
Iron (Fe) - 5.0% SIGNIFICANT DECREASE
Calcium (Ca) - 3.6%
Sodium (Na) - 2.8% SIGNIFICANT INCREASE
Potassium (K) - 2.6%
Magnesium (Mg) - 2.1% SIGNIFICANT DECREASE
The remaining elements, such as titanium, hydrogen, phosphorus, and trace elements, make up less than 1% of the crust's mass.
4. Rocks
The first rocks are believed to have formed in the early stages of the solar system, about 4.6 billion years ago, as the solar nebula began to cool and solidify. These rocks and minerals the size of space dust coalesced to form meteors, the planets, including Earth.
Geologists describe a rock as a naturally occurring, solid substance made up of one or more minerals. Rocks are classified based on their mineral composition, texture, and origin. The classical definitions of rock types are based on their origin and mineral composition. The three main categories of rocks are:
-
Igneous Rocks: These rocks are formed from the solidification of magma or lava. They can be further classified into intrusive igneous rocks, which cool and solidify below the Earth's surface, and extrusive igneous rocks, which cool and solidify on the Earth's surface. Examples of igneous rocks include granite, basalt, and pumice.
-
Sedimentary Rocks: These rocks are formed from the accumulation of sediment over time. Sediment can be derived from the weathering of pre-existing rocks or from the accumulation of organic material. Examples of sedimentary rocks include sandstone, limestone, and shale.
-
Metamorphic Rocks: These rocks are formed from the transformation of pre-existing rocks through heat, pressure, and chemical processes. Metamorphism can occur due to changes in temperature and pressure within the Earth's crust or due to contact with igneous intrusions. Examples of metamorphic rocks include marble, slate, and gneiss.
What type of rock is a Meteorite?
What type of rock is a Meteorite?
-
Some meteorites can be considered igneous rocks, as they have solidified from molten rock (magma or lava) at some point in their history.
For example, achondrite meteorites, which are typically composed of basaltic or granitic rock, are believed to have originated from volcanic activity on small asteroids or planetesimals in the early solar system. These rocks were then ejected into space through impacts and eventually made their way to Earth as meteorites.
Similarly, some stony-iron meteorites, such as pallasites, are thought to have formed from the mixing of molten metal and silicate rock in the cores of small planetary bodies. These rocks solidified and cooled over time, eventually being ejected into space and falling to Earth as meteorites.
-
Not all meteorites are considered metamorphic rocks, as they can come from a variety of sources and have different origins.
Some meteorites, particularly stony meteorites, are primitive and relatively unaltered, and thus have not undergone significant metamorphism. These meteorites may be composed of minerals that have remained largely unchanged since they first formed in the early solar system.
Other meteorites, particularly iron meteorites, are thought to have formed through processes such as melting and differentiation, rather than through metamorphism. These meteorites may have been partially or completely melted and re-solidified, but without undergoing the metamorphic processes that transform one rock type into another.