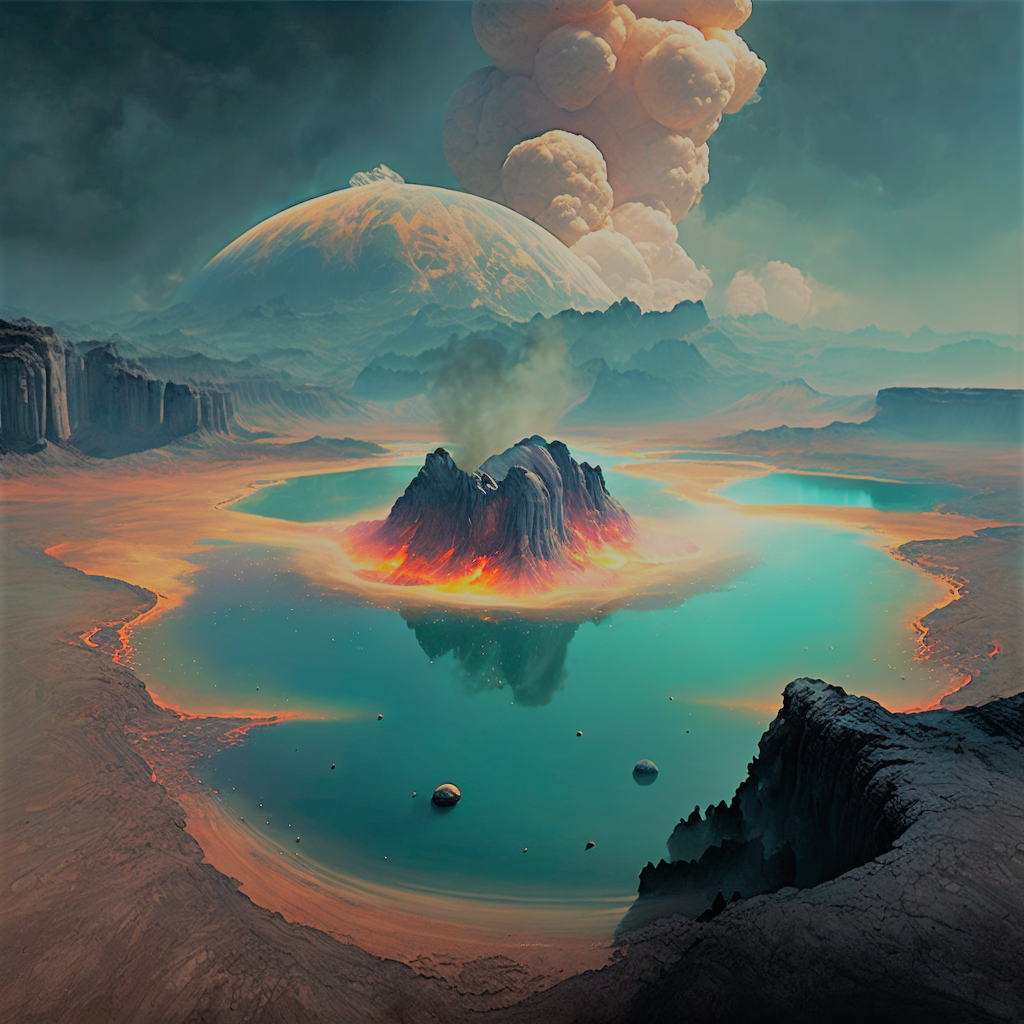
Chapter 6: The Archean Eon
First Lands and Seas
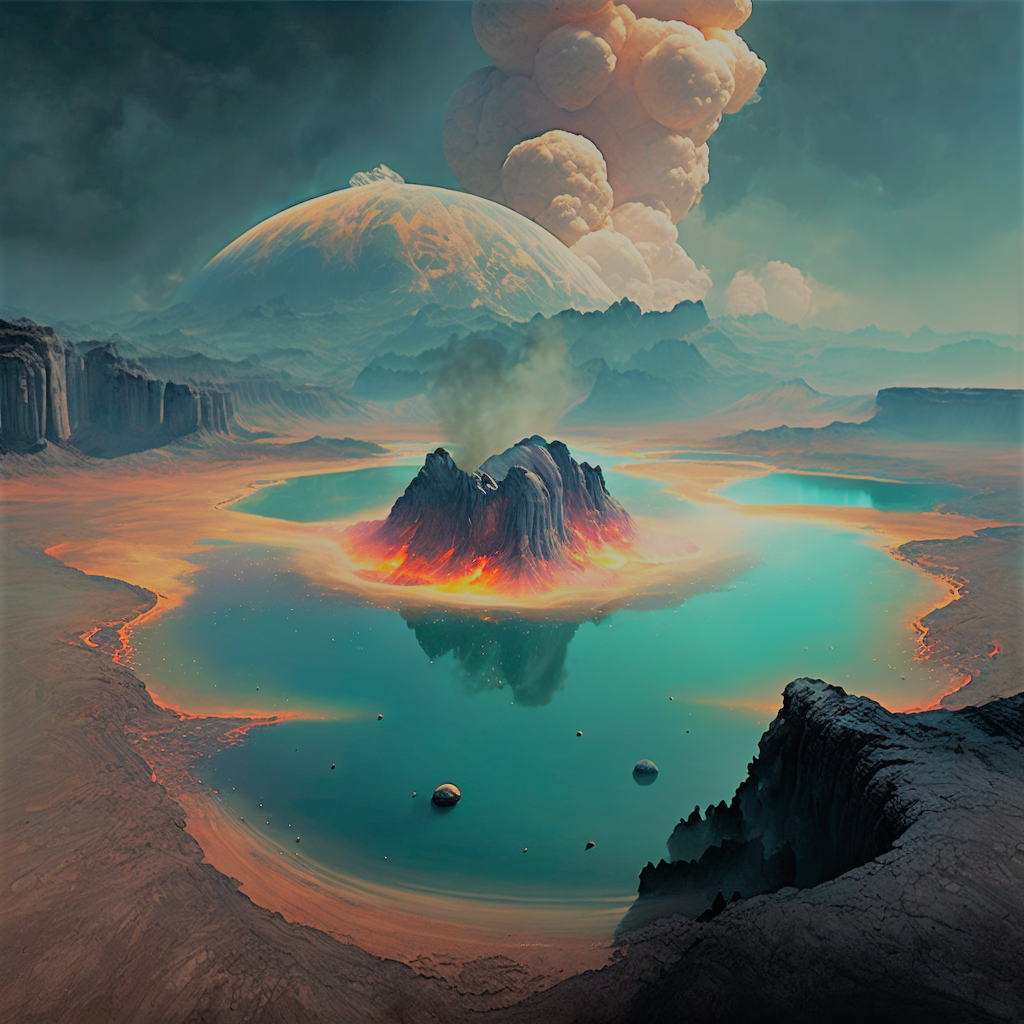
1. Archean
-
The Archean Eon is a crucial period in the history of the Earth, during which many of the key features of the planet were established. These include the formation of continental crust, the expansion of vast seas, the evolution of the atmosphere and the beginnings of life.
During the Archean Eon, the Earth was still undergoing intense volcanic and tectonic activity forming topographic highs above the surrounding oceans.
-
The early Archean atmosphere was likely composed of gases such as carbon dioxide (CO2), water vapor (H2O), nitrogen (N2), and methane (CH4), as well as small amounts of other gases such as hydrogen (H2), ammonia (NH3), and sulfur dioxide (SO2). The exact composition of the Archean atmosphere is still debated by scientists, but it is generally believed to have been much denser, hotter and more acidic than our modern atmosphere.
The Archean atmosphere is believed to have been much denser, hotter and more acidic than our modern atmosphere.
The Archean atmosphere is believed to have been much denser, hotter and more acidic than our modern atmosphere.
2. Liquid Water
Eventually, the exterior of the Earth cooled enough for a crust to form over the magma oceans that dominated the surface. At these temperatures liquid water could condense out of the hot and steamy atmosphere and pool in the low lying regions. Researchers disagree about the exact temperature of the early oceans, but it was likely much warmer than ours, with the same variation at the poles.
-
Very old zircon crystals within a rock in Western Australia have evidence of liquid water based on isotopic analysis of Oxygen molecules trapped within the crystal structure of the mineral.
The region in Australia is called the Jack Hills and the zircon crystals found there have been dated to be as old as 4.4 billion years.
-
Zircons are tiny, resilient mineral crystals primarily made of zirconium silicate (ZrSiO4). They are incredibly resistant to weathering and can be found in various geological formations, including some of the Earth's oldest rocks.
Zircon is valued for its ability to incorporate trace elements such as uranium, thorium, and hafnium into its crystal lattice, which makes it a useful tool for radiometric dating and for studying the geological history of the Earth.
One of the most important inclusions in these zircons is a specific isotopic composition of oxygen used to study the ancient atmosphere.
-
Oxygen has three naturally occurring isotopes: 16O, 17O, and 18O. The ratio of 18O to 16O is particularly useful in determining the history of water on Earth. This is because water containing 18O is slightly heavier than water containing 16O, and these isotopes fractionate, or separate, under different conditions. For example, during the process of evaporation, lighter 16O is preferentially evaporated, leaving behind water enriched in 18O.
The ancient zircons have a high ratio of 18O to 16O, which suggests that they formed in the presence of liquid water. This is because the zircons incorporated oxygen from the surrounding environment when they crystallized, and the high 18O/16O ratio indicates that the environment was likely influenced by the presence of liquid water. The presence of liquid water is further supported by the mineral inclusions within the zircons, which suggest that they formed under low-temperature and wet conditions.
-
It is also believed that the early ocean and atmosphere contained a much higher quantity of hydrogen compared to today.
-
An isotope is a variant of a chemical element that has the same number of protons but a different number of neutrons in its atomic nucleus. Since the number of protons determines the element's identity (and its place in the periodic table), all isotopes of a given element have the same chemical properties. However, they differ in mass due to the different numbers of neutrons.
For example, the most common isotope of oxygen is oxygen-16 (16O), which has eight neutrons (8 protons + 8 neutrons = 16). Another isotope of oxygen is oxygen-18 (18O), which has ten neutrons (8 protons + 10 neutrons = 18). Both oxygen-16 and oxygen-18 are isotopes of oxygen but have different atomic masses due to the differing numbers of neutrons in their nuclei.
There is also a third isotope of oxygen, oxygen-17 (17O), which has nine neutrons (8 protons + 9 neutrons = 17).
Isotopes can be either stable or unstable (radioactive). In the case of oxygen, all three isotopes mentioned above (16O, 17O, and 18O) are stable and do not undergo radioactive decay. Stable isotopes are often used in various scientific disciplines, such as geochemistry and paleoclimatology, to study environmental processes and conditions in the past.
-
Hydrogen-1 (protium, ¹H)
Hydrogen-2 (deuterium, ²H)
Helium-3 (³He)
Helium-4 (⁴He)
Lithium-6 (⁶Li)
Lithium-7 (⁷Li)
Beryllium-9 (⁹Be)
Boron-10 (¹⁰B)
Boron-11 (¹¹B)
Carbon-12 (¹²C)
Carbon-13 (¹³C)
Nitrogen-14 (¹⁴N)
Nitrogen-15 (¹⁵N)
Oxygen-16 (¹⁶O)
Oxygen-17 (¹⁷O)
Oxygen-18 (¹⁸O)
Fluorine-19 (¹⁹F)
Neon-20 (²⁰Ne)
Neon-21 (²¹Ne)
Neon-22 (²²Ne)
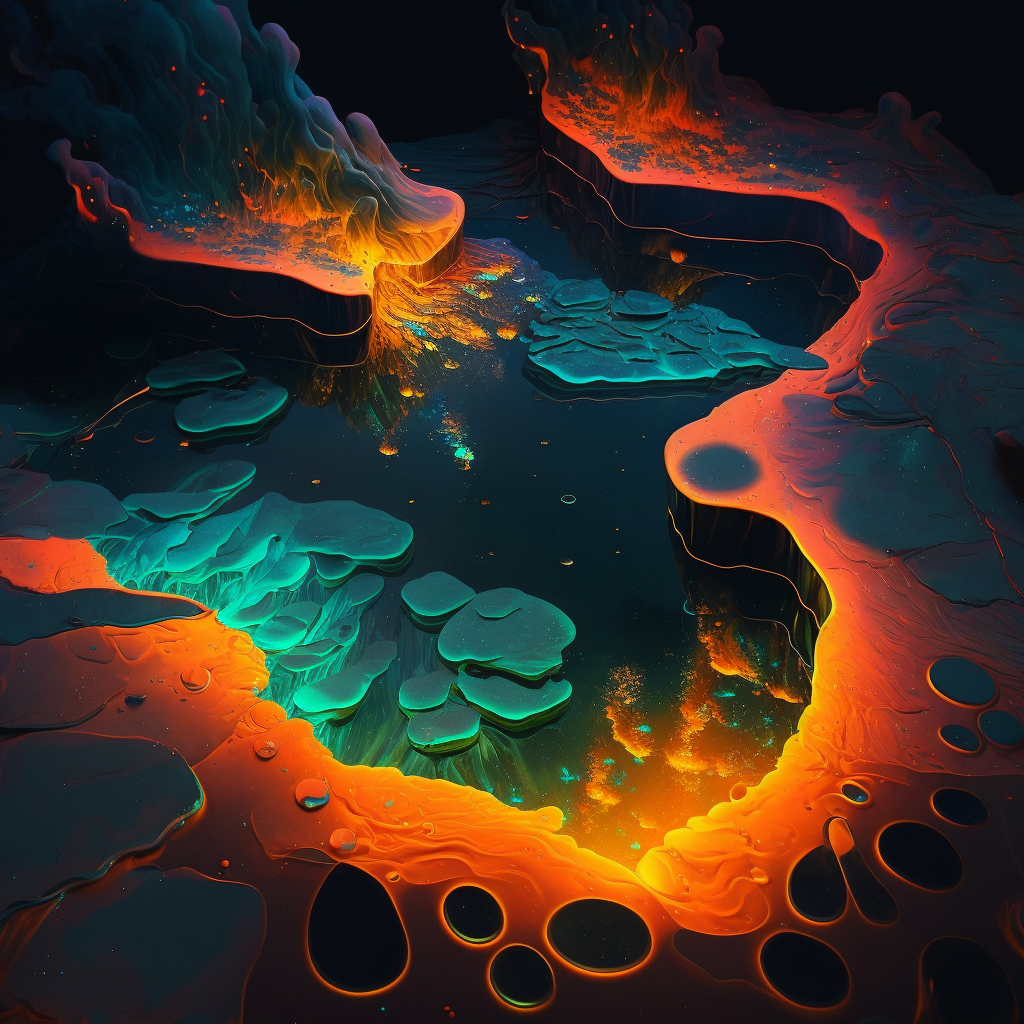
AI concept art of Early Archean pools of liquid water collecting in basins formed by the cooling outer crust. Inspiration was taken from hot springs to visualize the exotic environment.
-
The origin of Earth's water is still a topic of active research and debate among scientists, but there are several theories about where it may have come from.
-
The comet theory of water on Earth suggests that water was delivered to our planet by comets and other icy bodies during the early stages of the Solar System's formation. This theory is based on the observation that comets are made up of a variety of volatile compounds, including water, carbon dioxide, and methane, among others.
According to this theory, comets and other icy bodies may have collided with the young Earth and deposited water and other volatile compounds, which eventually accumulated in the planet's atmosphere and oceans. This process is thought to have taken place during the Late Heavy Bombardment period, which occurred around 4 billion years ago and involved a large number of impacts from comets and asteroids.
One piece of evidence in support of the comet theory is the fact that the ratio of deuterium to hydrogen in Earth's oceans is very similar to the ratio found in comets. This suggests that comets may have played a significant role in delivering water to our planet.
Additionally, studies of the isotopic composition of carbon, nitrogen, and other elements in comets and in the Earth's atmosphere and oceans have found similarities, further supporting the idea that comets may have contributed to the formation of our planet's water.
While the comet theory of water on Earth provides a compelling explanation for the presence of water on our planet, it is important to note that it is still a subject of ongoing scientific investigation. Other theories, such as the volcanic theory, also offer possible explanations for the origin of water on Earth.
-
Another theory is that the water on Earth was created through chemical reactions in the early solar system. In particular, reactions between hydrogen and oxygen in the solar nebula could have produced water. This water would have been gathered up with the gas and dust that formed the early earth.
The processes of plate tectonics and the resulting volcanic outgassing moved water from the interior of the earth to the surface as vapor and steam.
This theory is supported by several lines of evidence. For example, the Earth's mantle is believed to contain a large amount of water, which is stored in mineral structures such as serpentine and amphibole. These minerals are stable at high pressures and temperatures, and are thought to release water when they break down during volcanic activity.
Additionally, studies of volcanic rocks from around the world have found evidence of water and other volatile compounds such as carbon dioxide and sulfur dioxide. These compounds are typically released during volcanic eruptions and can contribute to the formation of new oceans and other bodies of water on the planet's surface.
One challenge to the volcanic theory of water on Earth is the fact that water is a relatively heavy molecule, and it is unclear how it would have been able to rise up from the Earth's interior to the surface. However, recent studies have suggested that the process of plate tectonics, which involves the movement of the Earth's crust and mantle, may have played a role in transporting water and other volatile compounds from the mantle to the surface.
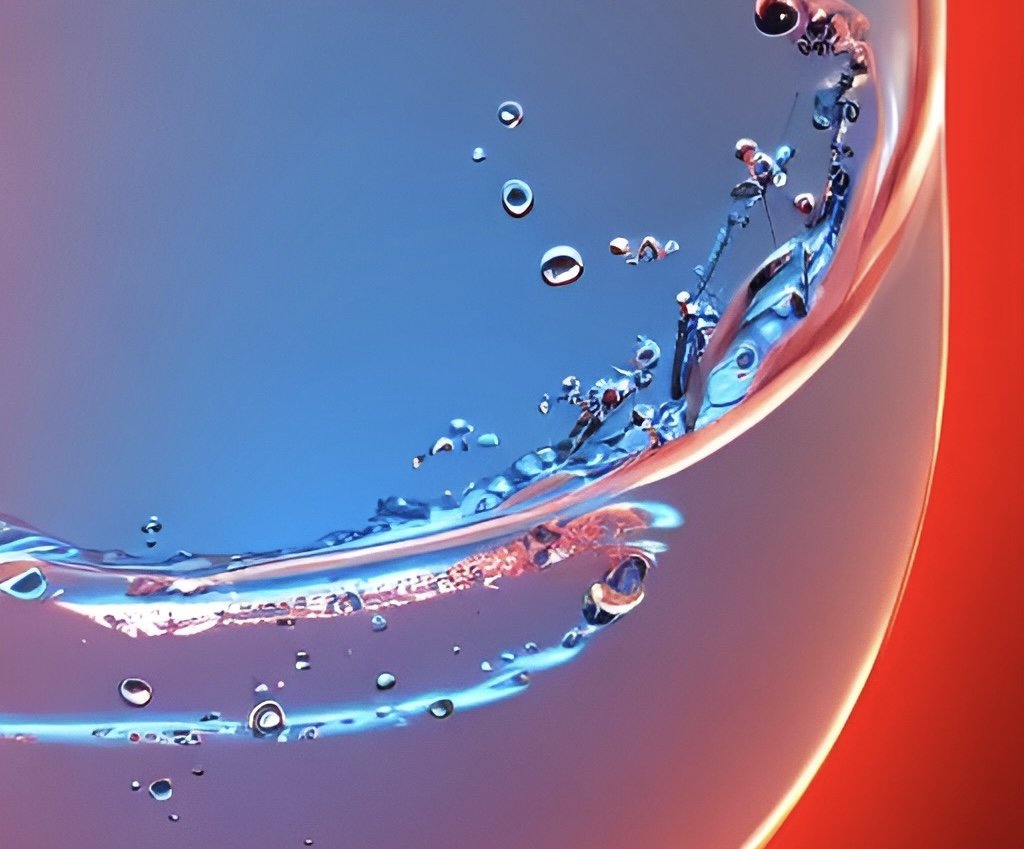
-
Earth, the Moon, and Mars have experienced different evolutionary histories and conditions, only Earth retained significant amounts of water through modern times.
The Moon and Mars lost most of their water over time. However, it is important to note that Mars still has substantial amounts of water in the form of ice at its poles and beneath its surface. The Moon also has water ice in permanently shadowed regions within craters near its poles.
-
Earth has a stronger gravitational pull and a much thicker atmosphere compared to the Moon and Mars. These factors help retain water and other volatile compounds on the surface and within the atmosphere. The Moon, with its minimal gravity and virtually no atmosphere, is unable to hold water on its surface. Mars, having a thin atmosphere and lower gravity, has also lost much of its surface water over time.
-
Earth has a strong, protective magnetic field generated by its molten iron core. This magnetic field shields the planet from solar wind, a stream of charged particles emitted by the Sun. Without this protection, solar wind can strip away the atmosphere and water from a planet's surface. Mars once had a magnetic field, but it weakened and disappeared billions of years ago, making the planet more susceptible to atmospheric loss due to solar wind. The Moon lacks a significant magnetic field, further contributing to its inability to retain water.
-
Earth is geologically active, with processes like volcanism, plate tectonics, and mantle convection constantly cycling water and other volatile compounds between the surface and the interior. This activity helps maintain Earth's water cycle and stabilize the planet's climate. The Moon and Mars, in contrast, have lower levels of geological activity. Mars may have had a more Earth-like water cycle in the past, but the decline in its geological activity over time has led to a decrease in water on its surface.
-
Earth is situated within the habitable zone, or the "Goldilocks zone," around the Sun, where temperatures are just right for liquid water to exist. The Moon, being a satellite of Earth, is also located in this zone, but its lack of atmosphere and low gravity prevent it from retaining water. Mars lies at the outer edge of the habitable zone and has experienced periods of warming and cooling throughout its history. These temperature fluctuations, combined with its thin atmosphere, have caused the majority of its water to either freeze or evaporate into space.
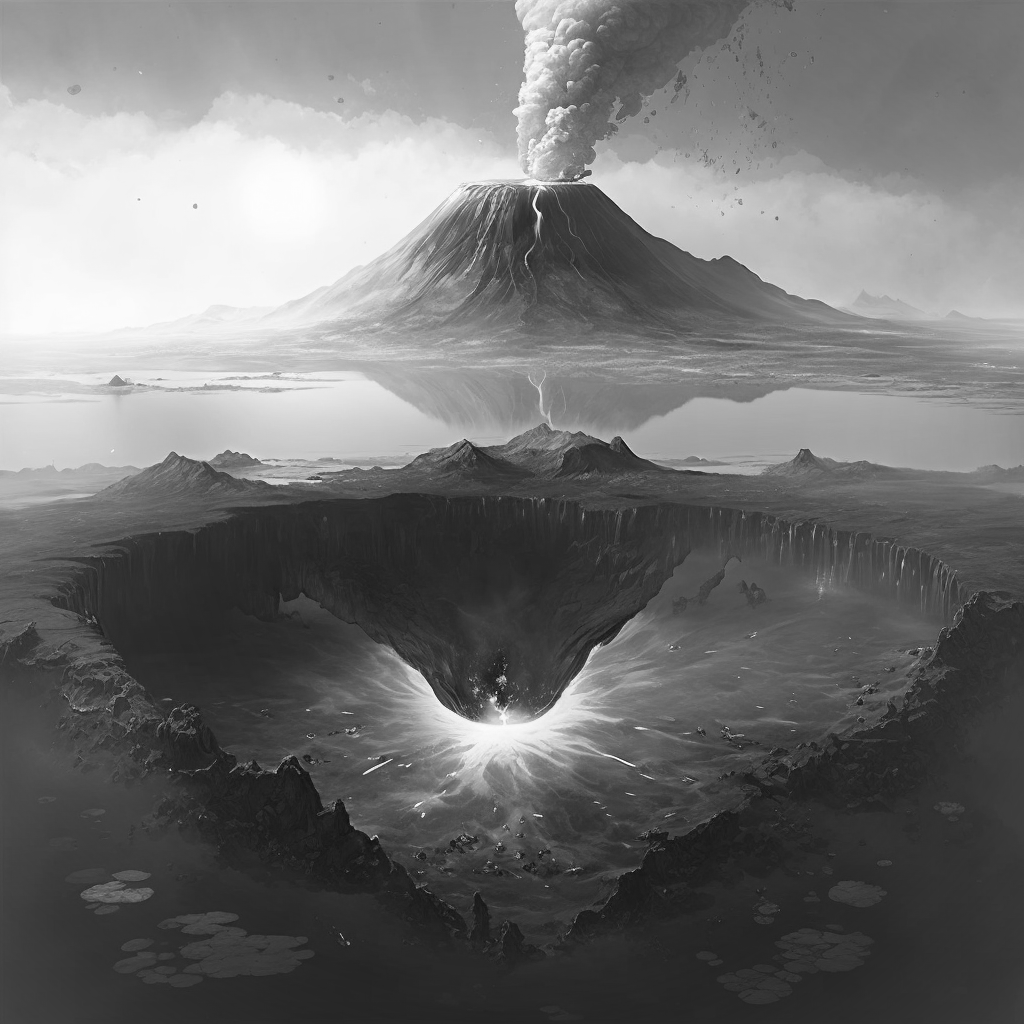
3. First Dry Land
The Earth’s Crust comes in two forms: Thick and Thin. Continental crust is thick, it has accumulated over time because it tends to remain buoyant, always “floating” on top like oil on water. Oceanic crust is thin and is in a constant state of formation and destruction due to plate tectonics.
The first continental crust was likely composed of a variety of rock types, including granite and tonalite-trondhjemite-granodiorite (TTG) suites. These rocks have a lower density than the basaltic rocks that make up the oceanic crust, allowing them to "float" on the denser mantle material and form the continents. The formation of the first continental crust is a topic of ongoing research and debate among geologists, but several key aspects can be highlighted
AI concept art illustrating a region of cooling magmas of different compositions, separating and solidifying to form early bits of the first Contintinental Crust.
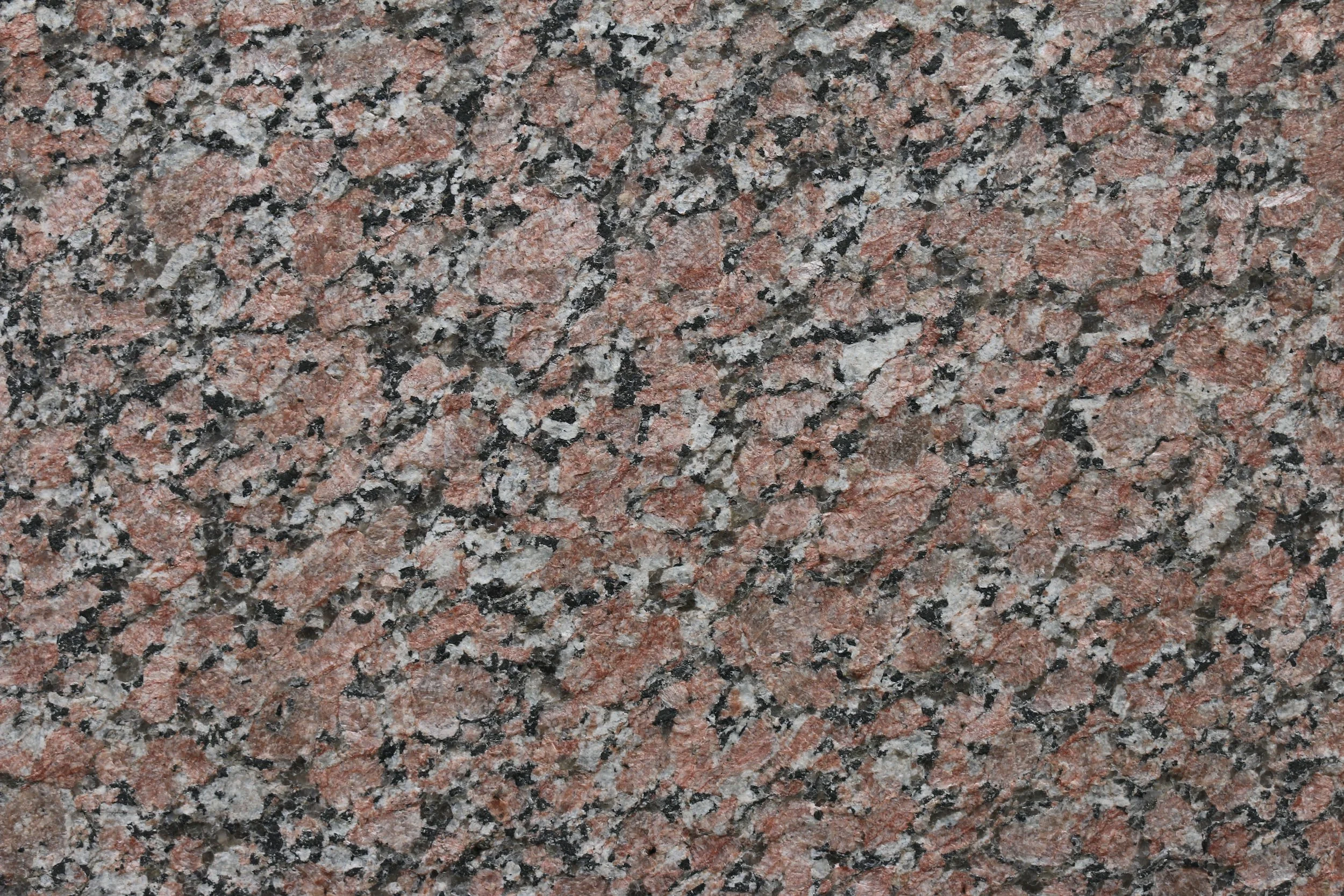
Half Dome is a dome-shaped granite rock formation located in Yosemite National Park in California, USA. Granite is a felsic, intrusive igneous rock that is formed when magma slowly cools and solidifies beneath the Earth's surface.
-
Continental crust is the thick, outermost layer of the Earth.
Composition: Continental crust is primarily composed of granitic rocks, which are light-colored, coarse-grained igneous rocks that are rich in aluminum and silicon. It also contains minor amounts of other minerals, including quartz, feldspar, and mica.
Structure: The crust is made up of several layers of different types of rocks, including sedimentary, igneous, and metamorphic rocks. These typically sit on large areas of ancient rock, known as cratons, that have been stable for billions of years.
Thickness: Continental crust is much thicker than oceanic crust, ranging from 30-50 kilometers in thickness on average. This is due to the fact that continental crust is less dense than oceanic crust, which allows it to float higher on the mantle.
Age: The oldest continental crust is over 4 billion years old, making it much older than oceanic crust. This is because continental crust is more stable and can survive for longer periods of time than oceanic crust.
Geological activity: Continental crust is less prone to volcanic and seismic activity than oceanic crust. However, it is still subject to deformation and uplift due to tectonic processes, which can create mountains, rift valleys, and other geological features.
Formation: Continental crust is formed through several processes, including the collision and merging of smaller landmasses, the uplifting of oceanic crust through tectonic processes, and the accumulation of sediment on the continental shelf.
-
"Felsic" is used to describe igneous rocks that are primarily composed of feldspar and silica minerals, and have a lower content of iron and magnesium. The term "felsic" comes from the German word "Feldspat," which means feldspar.
High silica content: Felsic magma, minerals, and rocks have a high silica content, typically greater than 65%, which makes them viscous and thick in texture.
Light color: Felsic minerals and rocks are typically light-colored, ranging from white to pink or red, due to the presence of quartz and feldspar minerals.
Low density: Felsic rocks have a relatively low density due to their low content of heavy minerals such as iron and magnesium.
Coarse-grained texture: Felsic rocks are often formed by slow cooling and solidification of magma, resulting in a coarse-grained texture with visible crystals.
High melting point: Felsic minerals and rocks have a high melting point due to their high silica content, which makes them resistant to melting and able to withstand high temperatures.
Brittle: Felsic rocks are generally more brittle than mafic rocks due to their low content of iron and magnesium.
Resistant to weathering: Felsic rocks are more resistant to weathering and erosion compared to mafic rocks due to their hardness and low iron and magnesium content.
FELSIC = High FELdspar and SIliCa, Low Fe & Mg
The Feldspar Group
-
Feldspars are a group of rock-forming minerals that constitute more than 60% of the Earth's crust, making them the most abundant mineral group on our planet. They are alumino-silicate minerals, composed of aluminum, silicon, and oxygen atoms, along with various metal ions such as potassium (K), sodium (Na), and calcium (Ca).
Feldspars can be divided into two main subgroups: alkali feldspars and plagioclase feldspars.
-
Alkali feldspars primarily contain potassium and sodium ions. The main end-members of this group are orthoclase (KAlSi3O8), which contains potassium, and albite (NaAlSi3O8), which contains sodium. Alkali feldspars exhibit a range of colors, from white to pink, depending on their composition and the presence of trace elements. They are commonly found in igneous rocks like granite and syenite, as well as in metamorphic rocks like gneiss and schist.
-
Plagioclase feldspars form a continuous series of compositions between albite (NaAlSi3O8) and anorthite (CaAl2Si2O8). They are characterized by the substitution of sodium and calcium ions within the crystal lattice. Plagioclase feldspars are common constituents of various rock types, including igneous rocks like basalt, andesite, and diorite, as well as metamorphic rocks like gneiss and schist.
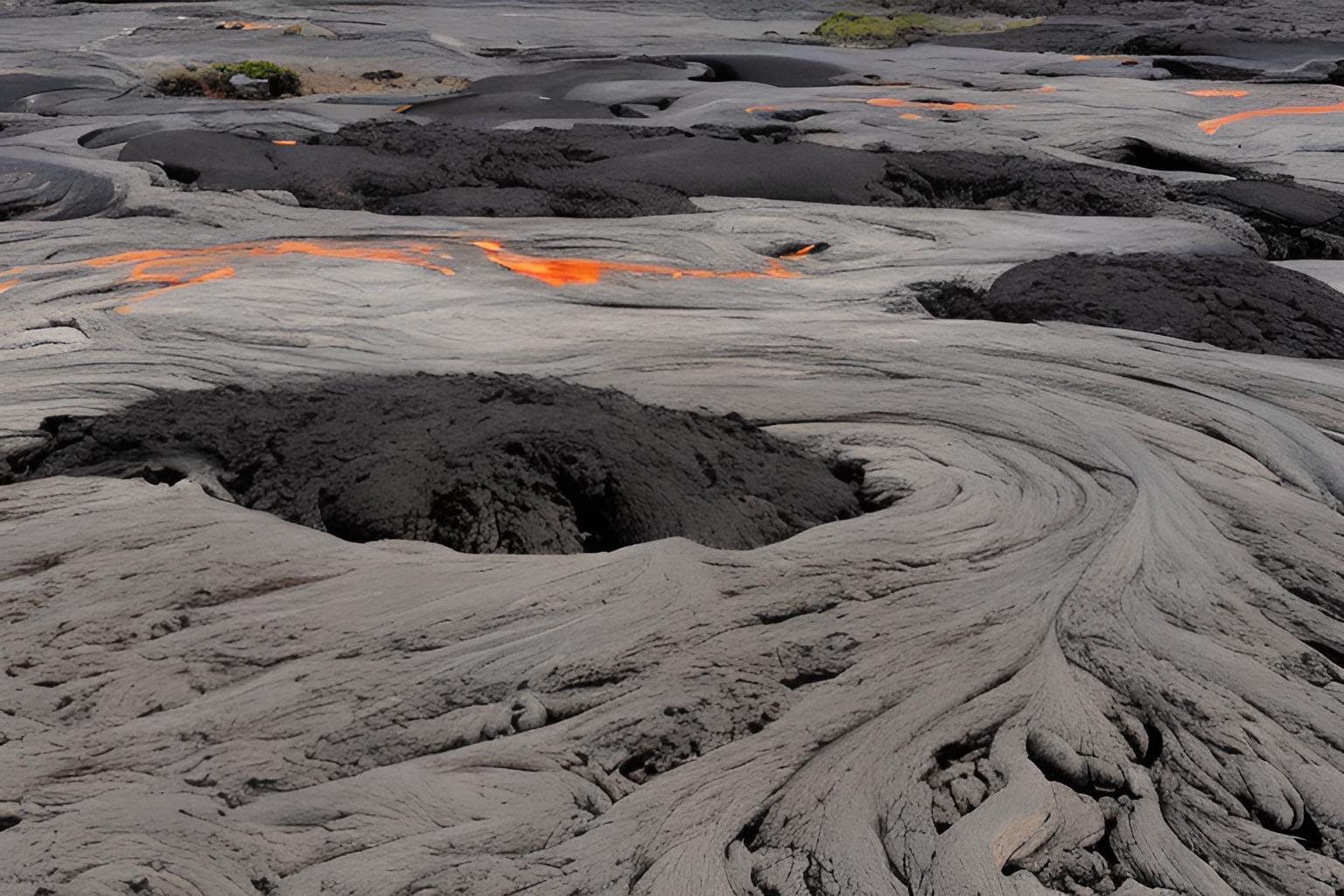
-
Oceanic crust is the thin layer of the Earth's crust that underlies the world's oceans. It is distinct from continental crust in its composition, structure, and thickness.
Composition: Oceanic crust is primarily composed of basaltic rocks, which are dark-colored, fine-grained volcanic rocks that are rich in iron and magnesium. It also contains minor amounts of other minerals, including olivine, pyroxene, and plagioclase feldspar.
Structure: Oceanic crust is layered, with a thin layer of sediment covering the basaltic rock. The sediment is primarily composed of fine-grained particles of clay, silt, and sand, which have been transported to the ocean floor by rivers, wind, and ocean currents.
Thickness: Oceanic crust is relatively thin, typically measuring 5-10 kilometers in thickness. This is in contrast to continental crust, which can be up to 50 kilometers thick.
Age: Oceanic crust is much younger than continental crust, with an average age of less than 200 million years. This is due to the fact that the oceanic crust is constantly being created at mid-ocean ridges and destroyed at subduction zones, whereas continental crust is more stable and can survive for billions of years.
Geological activity: Oceanic crust is associated with more volcanic and seismic activity than continental crust. This is because the oceanic crust is thinner and more malleable than the thicker, more rigid continental crust.
Formation: Oceanic crust is created at mid-ocean ridges, where magma rises from the mantle and solidifies to form new crust. As the crust moves away from the ridge, it cools and becomes denser, eventually sinking back into the mantle at subduction zones.
Overall, the fundamentals of oceanic crust are related to its composition, structure, thickness, age, and formation. These characteristics have important implications for the evolution and behavior of the Earth's crust, as well as for the development of natural resources such as minerals and oil.
-
"Mafic," on the other hand, is used to describe rocks that are primarily composed of magnesium and iron minerals, and have a lower content of silica. The term "mafic" comes from the first letters of the words "magnesium" and "ferric," which is an archaic term for iron.
Mafic magma, minerals, and rocks share some common properties due to their low silica content and high iron and magnesium content:
Low silica content: Mafic magma, minerals, and rocks have a low silica content, typically less than 52%, which makes them less viscous and more fluid in texture.
Dark color: Mafic minerals and rocks are typically dark-colored, ranging from black to dark gray, due to the presence of iron and magnesium minerals.
High density: Mafic rocks have a relatively high density due to their high content of heavy minerals such as iron and magnesium.
Fine-grained texture: Mafic rocks are often formed by rapid cooling and solidification of lava, resulting in a fine-grained texture with no visible crystals.
Low melting point: Mafic minerals and rocks have a lower melting point than felsic minerals and rocks, which makes them more susceptible to melting and able to melt at lower temperatures.
Ductile: Mafic rocks are generally more ductile than felsic rocks due to their high content of iron and magnesium.
Susceptible to weathering: Mafic rocks are more susceptible to weathering and erosion compared to felsic rocks due to their softer texture and higher content of iron and magnesium.
Examples of mafic minerals include olivine, pyroxene, and amphibole. Examples of mafic rocks include basalt, gabbro, and peridotite. These rocks are typically found in areas of recent volcanic activity such as mid-ocean ridges, island arcs, and hot spots. Mafic rocks have a wide range of uses in construction, including as aggregates and road building materials, as well as in the manufacturing of steel and other metals.
Columnar basalt in Iceland is formed by the cooling and solidification of lava flows that erupted from underwater volcanoes while forming new oceanic crust. The rapid cooling and contraction of the lava, combined with the effects of wave erosion and other geological forces, have resulted in the distinctive hexagonal columns that make up the basalt formations.
MAFIC = MAgnesium and FerrIC (the old term for iron)
-
Weathering is the process by which rocks, minerals, and soils are broken down and transformed by exposure to the Earth's atmosphere, water, and other natural processes. There are two main types of weathering: mechanical weathering and chemical weathering.
Weathering is an important geological process that contributes to the formation and evolution of landscapes, soils, and sedimentary rocks. It also plays a critical role in the carbon cycle and the cycling of other elements on Earth, helping to regulate the chemistry and composition of the atmosphere, oceans, and biosphere.
-
Mechanical weathering is the physical breaking down of rocks and minerals into smaller pieces. This can happen through a variety of processes, including freezing and thawing, the actions of plant roots and burrowing animals, and abrasion by wind, water, or other materials.
-
Chemical weathering is the breakdown of rocks and minerals through chemical reactions with the atmosphere, water, or other substances. This can happen through a variety of processes, including oxidation, hydrolysis, and dissolution. These chemical reactions can alter the composition and properties of rocks and minerals, often resulting in the formation of new minerals or compounds.
-
The interaction between the Archean atmosphere and the young crust of mafic and felsic material would have been complex and dynamic. The dense atmosphere, high levels of greenhouse gases, and lack of free oxygen would have influenced the composition and properties of the crust, while the properties of the crust would have influenced the chemistry and evolution of the atmosphere.
The mafic crust would have been more susceptible to weathering and erosion due to its softer texture and higher content of iron and magnesium. The weathering of mafic rocks would have released elements such as calcium, magnesium, and iron into the oceans, which could have contributed to the development of early life forms. Felsic crust, on the other hand, would have been more resistant to weathering and erosion due to its harder texture and lower iron and magnesium content.
The interaction between the atmosphere and the crust would have also influenced the carbon cycle on early Earth. The high levels of carbon dioxide in the atmosphere would have contributed to the formation of carbonic acid, which could have reacted with minerals in the crust to form carbonate rocks. This process would have removed carbon dioxide from the atmosphere, helping to regulate the climate and prevent a runaway greenhouse effect.
Overall, the interaction between the Archean atmosphere and the young crust of mafic and felsic material would have been complex and influenced the evolution of the Earth's climate, geology, and biology. The properties of the crust would have influenced the chemistry of the atmosphere, while the composition and properties of the atmosphere would have influenced the formation and evolution of the crust.
Main Processes of Mechanical Weathering
-
This occurs when rocks are exposed to extreme temperature changes, such as when they are heated by the sun during the day and then rapidly cooled at night. The expansion and contraction caused by these temperature changes can lead to the formation of cracks and other types of damage.
-
This occurs when rocks that have been buried deep in the Earth's crust are exposed by erosion or other processes. As the pressure on the rocks is released, they can expand and crack, leading to the formation of fractures and other types of damage.
-
This occurs when rocks are worn down by the action of wind, water, or other materials. For example, sand carried by wind or water can wear away at the surface of rocks over time.
-
This occurs when water seeps into cracks in rocks and then freezes, expanding as it does so. The expansion of the ice can cause the cracks to widen and eventually break the rock apart.
-
This occurs when plant roots grow into cracks in rocks and then expand, causing the cracks to widen and eventually break the rock apart.
Three Main Processes of Chemical Weathering
-
Hydrolysis involves the reaction of a mineral or compound with water (H2O) to form new compounds. This typically occurs through the breaking of chemical bonds in the mineral or compound. For example, feldspar minerals (such as potassium feldspar or KAlSi3O8) can react with water to form clay minerals (such as kaolinite or Al2Si2O5(OH)4) and other compounds. The chemical reaction can be represented as follows: KAlSi3O8 + 2H2O → Al2Si2O5(OH)4 + 4SiO2 + K+ + 2H+.
-
Dissolution occurs when a mineral or compound is dissolved in water or another solvent. This typically occurs when the mineral or compound is exposed to an acidic solution, which can break down the chemical bonds and cause the compound to dissolve. For example, when calcium carbonate (CaCO3) is exposed to acidic water, it can react to form calcium ions (Ca2+) and bicarbonate ions (HCO3-). The chemical reaction can be represented as follows: CaCO3 + H+ → Ca2+ + HCO3-.
-
The chemical formula for oxygen gas is O2. When oxygen reacts with other substances, it can gain electrons and form an oxide compound. In the case of rocks and minerals, this often results in the formation of iron oxide (Fe2O3) or other metal oxide compounds. For example, when iron (Fe) reacts with oxygen (O2), it forms iron oxide (Fe2O3) or rust.
4. The Oldest Rocks
The oldest rocks on Earth have experienced a range of weathering processes, such as chemical and physical weathering, as well as tectonic stresses like compression, tension, and shearing. These processes have caused rocks to crack and break apart, leading to the formation of faults and fractures, and the metamorphism of rocks and minerals under high temperature and pressure conditions. Over time, erosion from wind, water, and other natural processes has also contributed to the breakdown and transportation of rocks and minerals, leading to the formation of sedimentary rocks and other geological features.
-
Located in the Northwest Territories of Canada, the Acasta Gneiss is one of the oldest known rock formations on Earth, with an age of approximately 4.03 billion years. This rock is primarily composed of tonalite gneiss and is part of the Canadian Shield. The Acasta Gneiss provides insights into the processes of crust formation and the early Earth's geological evolution.
-
Found in Western Australia, the Narryer Gneiss Terrane contains zircon crystals that have been dated to around 4.4 billion years old. While the rocks themselves are not quite as old (with ages around 3.8 to 3.9 billion years), the presence of these ancient zircon crystals indicates that some of the material within the rocks was formed more than 4 billion years ago. The Narryer Gneiss Terrane provides information about the early Earth's crust and the conditions that existed during the Hadean Eon.
-
The ages of the oldest rocks on Earth are determined using radiometric dating techniques, which rely on the decay of unstable isotopes of elements such as uranium and potassium. By measuring the ratio of parent isotopes to daughter isotopes in a rock sample, scientists can calculate the age of the rock. Radiometric dating techniques such as uranium-lead dating and potassium-argon dating are commonly used to date rocks that are billions or millions of years old, respectively. Additionally, zircon dating can be used to determine the age of rocks by analyzing the age of zircon mineral grains within them.
-
Radiometric dating is a method used to determine the age of rocks and other geological materials based on the decay of radioactive isotopes. This technique relies on the fact that certain isotopes of elements are unstable and decay into other isotopes at a known rate, which is referred to as the "half-life" of the isotope.
The process of radiometric dating involves measuring the relative abundance of the parent and daughter isotopes in a rock or mineral sample. The parent isotope is the original radioactive isotope, and the daughter isotope is the product of the decay process. By measuring the ratio of the parent to daughter isotopes in a sample, scientists can calculate the age of the sample.
To carry out radiometric dating, scientists typically use a mass spectrometer to measure the isotopic ratios in a sample. This involves analyzing the sample's composition, separating the isotopes of interest, and then measuring their relative abundance.
Radiometric dating can be used to determine the age of a variety of geological materials, including rocks, minerals, and fossils. Different isotopes have different half-lives, which makes them useful for dating materials of different ages. For example, carbon-14 dating is used to date materials that are up to 50,000 years old, while uranium-lead dating can be used to date rocks that are billions of years old.
One important consideration when using radiometric dating is the potential for contamination. It is important to ensure that the sample being analyzed is free from any extraneous material that could affect the isotopic ratios. Additionally, some isotopes have more than one decay pathway, which can make the dating process more complex.
Despite these challenges, radiometric dating is a powerful tool for understanding the age and evolution of the Earth and other objects in the universe.
-
Uranium-lead dating is a radiometric dating technique used to determine the age of rocks and minerals that are billions of years old. It is based on the decay of uranium isotopes to lead isotopes, which occurs at a known rate over time. Specifically, uranium-238 decays to lead-206, while uranium-235 decays to lead-207.
To determine the age of a rock or mineral using uranium-lead dating, scientists measure the ratios of parent uranium isotopes to daughter lead isotopes present in the sample. They do this using a mass spectrometer, which separates and measures the isotopes based on their mass and charge. By comparing the measured ratios of uranium and lead isotopes to the known decay rates of the uranium isotopes, scientists can calculate the age of the sample.
Uranium-lead dating is a highly accurate dating method, and is commonly used to date rocks and minerals that are hundreds of millions to billions of years old. It is particularly useful for dating zircon minerals, which are highly resistant to weathering and can often survive as tiny crystals within older rocks.
One limitation of uranium-lead dating is that it is susceptible to errors caused by the loss or gain of lead or uranium isotopes due to geological processes such as weathering, metamorphism, or melting. Additionally, the technique is expensive and time-consuming, requiring careful sample preparation and analysis. Despite these limitations, uranium-lead dating remains an important tool for understanding the ages and histories of rocks and minerals.
-
Half-life is the time it takes for half of the radioactive isotopes in a sample to decay into stable daughter isotopes. Radioactive decay is a random process, meaning that the rate of decay cannot be predicted with certainty. However, scientists have found that for a given radioactive isotope, the rate of decay is proportional to the number of parent isotopes present in the sample.
For example, if a sample of a radioactive isotope has a half-life of one hour, then after one hour, half of the parent isotopes in the sample will have decayed into daughter isotopes. After two hours, half of the remaining parent isotopes will have decayed, leaving only one-quarter of the original parent isotopes in the sample. After three hours, half of the remaining parent isotopes will have decayed, leaving only one-eighth of the original parent isotopes in the sample, and so on.
The concept of half-life is important in radiometric dating, which is used to determine the age of rocks, minerals, and other geological materials. By measuring the relative abundance of parent and daughter isotopes in a sample, scientists can calculate the number of half-lives that have passed since the sample was last heated or exposed to high temperatures. By multiplying the number of half-lives by the half-life of the isotope, scientists can determine the age of the sample.
Different radioactive isotopes have different half-lives, which makes them useful for dating materials of different ages. For example, carbon-14 dating is used to date materials that are up to 50,000 years old, while uranium-lead dating can be used to date rocks that are billions of years old.
Overall, the concept of half-life is a fundamental concept in the field of radiochemistry and is crucial for understanding the behavior of radioactive isotopes in the natural world.
-
A mass spectrometer is an instrument used to measure the mass-to-charge ratio of ions in a sample. The basic operation of a mass spectrometer involves five main steps:
Ionization: The sample is first ionized, which means that the atoms or molecules in the sample are converted into ions. This is typically done by bombarding the sample with high-energy particles such as electrons or by subjecting it to a strong electric field.
Acceleration: The ions are then accelerated by an electric field, which causes them to gain kinetic energy and move towards a detector.
Separation: The ions are separated based on their mass-to-charge ratio (m/z) using a magnetic field. The magnetic field causes the ions to follow a curved path, with ions of different masses following different paths. This separation is based on the principle that the radius of the path followed by an ion is proportional to its mass-to-charge ratio.
Detection: The separated ions are detected using a detector, which produces a signal proportional to the number of ions that reach it. The detector can be a simple metal plate that measures the current produced by the ions or a more sophisticated device such as a microchannel plate or an electron multiplier.
Analysis: The data from the detector is analyzed using a computer, which can calculate the mass-to-charge ratio of the ions based on their separation in the magnetic field. The results are typically displayed as a mass spectrum, which shows the relative abundance of ions of different mass-to-charge ratios in the sample.
Modern mass spectrometers can be highly sophisticated instruments that incorporate a range of advanced technologies. For example, some mass spectrometers use time-of-flight (TOF) detection, which measures the time it takes for ions to travel a certain distance and calculates their mass-to-charge ratio based on this information. Other types of mass spectrometers, such as quadrupole mass spectrometers, use electric fields to separate ions of different mass-to-charge ratios.
Overall, mass spectrometry is a powerful analytical technique that has applications in a wide range of fields, including chemistry, biology, geology, and environmental science.
-
The history of radiochemistry dates back to the late 19th century, when scientists began to explore the properties of radioactive elements such as uranium, thorium, and radium. One of the pioneers of this field was the French physicist Henri Becquerel, who in 1896 discovered that certain materials could emit radiation without the need for external stimuli.
In 1898, the husband-and-wife team of Pierre and Marie Curie isolated the element radium and demonstrated that it gave off a constant stream of radiation. Their work laid the foundation for the field of radiochemistry, which would come to encompass the study of radioactive materials, their properties, and their applications in a range of fields, including medicine, industry, and nuclear energy.
Marie Curie's daughter, Irène Curie, also made significant contributions to the field of radiochemistry. Along with her husband, Frédéric Joliot, she discovered artificial radioactivity, which is the process of creating new, radioactive isotopes by bombarding stable elements with high-energy particles.
Throughout the 20th century, radiochemistry continued to evolve and expand, with new discoveries and applications in fields such as radiopharmaceuticals, nuclear medicine, and environmental science. The development of new techniques such as mass spectrometry and high-resolution imaging have enabled scientists to study the behavior of radioactive isotopes at the atomic and molecular level, providing new insights into the properties and behavior of matter.
Today, radiochemistry remains an important field of study with many applications in industry, medicine, and research. The study of radiochemistry is essential for understanding the behavior of radioactive materials in the natural world and for developing new technologies for the safe handling and disposal of radioactive waste.
n. Gneiss and Metamorphic Rocks
-
Metamorphic rocks are a type of rock that have been transformed from their original state through exposure to heat, pressure, and/or chemical activity. This transformation process can cause changes in the mineral composition, texture, and structure of the rock, resulting in new minerals and textures that are different from the original rock. Metamorphic rocks are typically formed from pre-existing rocks, which can be sedimentary, igneous, or other metamorphic rocks.
The process of metamorphism can occur over a wide range of temperatures and pressures, and can be influenced by a variety of factors such as the composition of the original rock, the duration of the metamorphic event, and the presence of fluids or other chemical agents. The type of metamorphic rock that forms depends on the specific conditions of metamorphism, as well as the original rock type and its mineral content.
There are two main types of metamorphism: regional metamorphism and contact metamorphism.
Regional metamorphism occurs over a large area and is typically associated with tectonic activity, such as the collision of continental plates or the subduction of one plate beneath another. Regional metamorphism can result in the formation of a wide range of metamorphic rocks, including schist, gneiss, and marble.
Contact metamorphism, on the other hand, occurs when rocks are exposed to high temperatures and pressures near a magma chamber. This type of metamorphism is typically localized and can result in the formation of rocks such as hornfels or skarn.
The type of metamorphic rock that forms depends on a variety of factors, including the temperature and pressure conditions, the original rock type and its mineral content, and the duration of the metamorphic event.
For example, the formation of slate requires relatively low temperatures and pressures, while the formation of gneiss requires high temperatures and pressures over an extended period of time.
Metamorphic rocks have a wide range of physical and chemical properties that make them useful in a variety of applications. For example, marble is a common building material due to its durability and aesthetic appeal, while slate is often used for roofing tiles and flooring due to its low porosity and resistance to weathering. Metamorphic rocks can also provide valuable insights into the geological history of an area, as they can provide clues about the temperature, pressure, and chemical conditions that existed during the metamorphic event.
-
Gneiss is a type of metamorphic rock that is formed from other rocks under conditions of high pressure and temperature. It is typically composed of alternating layers or bands of different minerals, giving it a distinctive striped appearance. The name "gneiss" comes from a German word meaning "sparkling" or "shining," which refers to the reflective quality of some of the minerals found in the rock.
Gneiss can form from a variety of parent rocks, including granite, shale, and volcanic rocks. The process of gneiss formation typically involves the recrystallization of minerals in the parent rock, as well as the development of new minerals as a result of the increased heat and pressure.
One of the most striking features of gneiss is its banding, which is often visible to the naked eye. This banding is caused by the segregation of different minerals into distinct layers or bands, which can be horizontal or tilted at an angle. The mineral composition of these bands can vary widely, but common minerals found in gneiss include quartz, feldspar, mica, and hornblende.
Gneiss is a common rock type found throughout the world, and it has a wide range of applications in construction, sculpture, and other industries. Its durability and aesthetic appeal make it a popular choice for building facades, flooring, and other decorative uses.
-
The composition of the bands in gneiss can vary widely depending on the parent rock and the specific conditions of metamorphism. However, there are some common minerals that are often found in gneiss bands, including:
Quartz: a mineral that is very hard and resistant to weathering. It often forms white or gray bands in gneiss.
Feldspar: a group of minerals that are important components of many igneous rocks. They often form light-colored bands in gneiss.
Mica: a mineral that is characterized by its sheet-like structure and excellent cleavage. It often forms dark-colored bands in gneiss.
Hornblende: a mineral that is a common component of many metamorphic rocks. It often forms dark-colored bands in gneiss.
Other minerals that may be present in gneiss bands include garnet, staurolite, kyanite, and sillimanite, among others. The specific minerals present in the bands can provide clues about the conditions under which the gneiss formed, such as the temperature and pressure of the metamorphic process. The mineral composition of gneiss bands can also affect the rock's properties, such as its strength, durability, and resistance to weathering.
-
Quartz is a mineral that is composed of silicon and oxygen atoms arranged in a repeating pattern of tetrahedra. It is one of the most abundant minerals on Earth and can be found in a wide variety of geological environments. Quartz has a hardness of 7 on the Mohs scale, making it one of the hardest minerals known to exist.
Quartz is a common component of many types of rocks, including granite, sandstone, and quartzite. It is often translucent or transparent, and can range in color from clear or white to gray, brown, purple, pink, and other hues. The color of quartz is often influenced by impurities within the crystal lattice, such as iron, titanium, or other elements.
Quartz is valued for its physical and chemical properties, which make it useful in a wide variety of industrial applications. It is highly resistant to chemical weathering and erosion, which makes it an ideal material for use in construction, as well as for making glass, ceramics, and electronics. Quartz is also piezoelectric, meaning that it can generate an electric charge when subjected to pressure, and this property makes it useful for sensors, oscillators, and other electronic components.
In addition to its practical applications, quartz has long been valued for its beauty and is commonly used in jewelry and other decorative objects. Quartz crystals are often cut and polished into gemstones, and their unique optical properties can create dazzling effects such as color change or iridescence. Quartz is also a common mineral in many metaphysical and spiritual traditions, and is believed by some to have healing properties or other mystical properties.
-
Feldspar is a group of minerals that are among the most common minerals on Earth. They are composed of aluminum, silicon, and oxygen atoms arranged in a repeating framework of tetrahedra. Feldspar minerals typically form in igneous and metamorphic rocks and are also found in sedimentary rocks.
There are two main types of feldspar: potassium feldspar (also known as orthoclase) and plagioclase feldspar. Potassium feldspar is typically pink, red, or white in color, while plagioclase feldspar is typically gray or white. Both types of feldspar have a hardness of 6 on the Mohs scale, making them relatively hard minerals.
Feldspar minerals have a wide range of physical and chemical properties that make them useful in a variety of applications. For example, feldspar is commonly used in the manufacture of ceramics, glass, and pottery, where it serves as a fluxing agent that helps to lower the melting point of the raw materials. Feldspar is also used in the production of cement, where it serves as a source of aluminum and silica.
In addition to its industrial uses, feldspar is also prized for its beauty and is commonly used in jewelry and other decorative objects. Some varieties of feldspar, such as moonstone, labradorite, and sunstone, exhibit a phenomenon known as adularescence, which is a unique play of light caused by the internal structure of the crystal.
Feldspar minerals also have important geological implications. Because they are common components of many igneous and metamorphic rocks, their presence and distribution can provide clues about the origin and history of these rocks. For example, the presence of certain types of feldspar in a rock can help to determine the temperature and pressure conditions under which the rock formed.
-
Mica is a group of silicate minerals that are known for their unique physical properties. They are composed of aluminum, silicon, oxygen, and hydrogen atoms arranged in thin, flat layers. Mica minerals are characterized by their perfect cleavage, which allows them to be easily split into thin, flexible sheets. These sheets are transparent, translucent, or opaque and can range in color from white and colorless to shades of brown, green, and black.
Mica minerals are found in many types of rocks, including granite, gneiss, schist, and pegmatite. They are also found in soils and sediments, and are sometimes used as a geothermometer to determine the temperature history of rocks.
Mica minerals have a wide range of practical applications due to their unique physical properties. They are used as insulators in electrical and electronic equipment, as well as in construction materials such as drywall and cement. Mica is also used as a filler in paints and plastics, as a lubricant in drilling fluids, and as a component of cosmetics and personal care products.
Mica minerals are also prized for their beauty and are commonly used in jewelry and other decorative objects. Some varieties of mica, such as muscovite, exhibit a phenomenon known as pleochroism, which causes them to display different colors when viewed from different angles.
In addition to their practical and aesthetic uses, mica minerals also have important geological implications. Because they are common components of many types of rocks, their presence and distribution can provide valuable information about the origin and history of these rocks. For example, the presence of mica minerals in a rock can indicate the temperature and pressure conditions under which the rock formed, as well as the composition of the parent magma or sediment.
-
Hornblende is a mineral that is a member of the amphibole group of minerals. It is composed of calcium, magnesium, iron, aluminum, silicon, oxygen, and hydrogen atoms arranged in a complex crystal lattice. Hornblende is typically black or dark brown in color, although it can also be green or blue-green.
Hornblende is a common component of many types of igneous and metamorphic rocks, including basalt, diorite, and gneiss. It is also found in some sedimentary rocks, where it may have been transported as detrital grains.
Hornblende has a number of physical and chemical properties that make it useful in a variety of applications. For example, hornblende is a common source of iron and magnesium in the manufacture of iron and steel. It is also used as a flux in the production of glass and ceramics, where it helps to lower the melting point of the raw materials.
In addition to its practical uses, hornblende is also prized for its beauty and is commonly used in jewelry and other decorative objects. Some varieties of hornblende, such as tremolite, actinolite, and edenite, exhibit a unique fibrous or needle-like texture and are known as asbestos. Asbestos has been used for its fire-resistant properties in building materials and other products, but its use has been greatly reduced due to health concerns associated with inhaling the fibers.
Hornblende also has important geological implications. Because it is a common component of many types of rocks, its presence and distribution can provide valuable information about the origin and history of these rocks. For example, the presence of hornblende in a rock can indicate the temperature and pressure conditions under which the rock formed, as well as the composition of the parent magma or sediment.
“The formation of gneiss requires high temperatures and pressures over an extended period of time.”
-
The Acasta Gneiss is located in the Acasta River region of the Northwest Territories, Canada. It is one of the oldest known rocks on Earth, with an estimated age of 4.03 to 4.02 billion years old. The Acasta Gneiss is part of the Slave Province, which is a geological region that is characterized by its ancient rocks and complex tectonic history.
The Acasta Gneiss is composed of several minerals, including quartz, feldspar, and biotite. It has a medium- to coarse-grained texture, with a foliated or banded appearance that is typical of gneiss rocks. The gneiss is notable for its high degree of metamorphism, which is thought to have been caused by intense heat and pressure during the early stages of the Earth's formation.
The Acasta Gneiss is important to geologists and researchers because it provides valuable insights into the early history of the Earth. The gneiss is believed to have formed during the Hadean Eon, which is the earliest known period in Earth's history, when the planet was still cooling and its crust was forming. The age of the Acasta Gneiss makes it one of the only known rocks on Earth that has survived from this time period, and it provides valuable information about the geological and environmental conditions that existed at that time.
In addition to its scientific importance, the Acasta Gneiss is also an important cultural and spiritual site for indigenous peoples in the region. The rock formation is regarded as a sacred place by the local Dene and Métis communities, who have long-standing cultural and spiritual connections to the land.
CONTINENTAL CRUST
Oldest remnant of early continental crust - Zircon crystals within the Narryer Gneiss (type of metamorphic rock) in the Jack Hills region of Western Australia. There is a lot of speculation about the composition of the early crust. The crust is cool and brittle and sits over the hotter plastic-like asthenosphere
Continental crust - Felsic, lighter, floats on heaver material
Cratons - The early continents
Erosion - Chemical & Physical due to the movement of the water cycle. Mountains reach equilibrium with the atmosphere, the rate of erosion at the peak of Everest matches the rate of growth due to the collision of plate boundaries
Oldest Sedimentary Rocks - 3.8 Billion Years old - Isua Supracrustal rocks from Greenland
ArcGIS
* BONUS *
ArcGIS * BONUS *
Virtual Reality
* BONUS *
Virtual Reality * BONUS *
