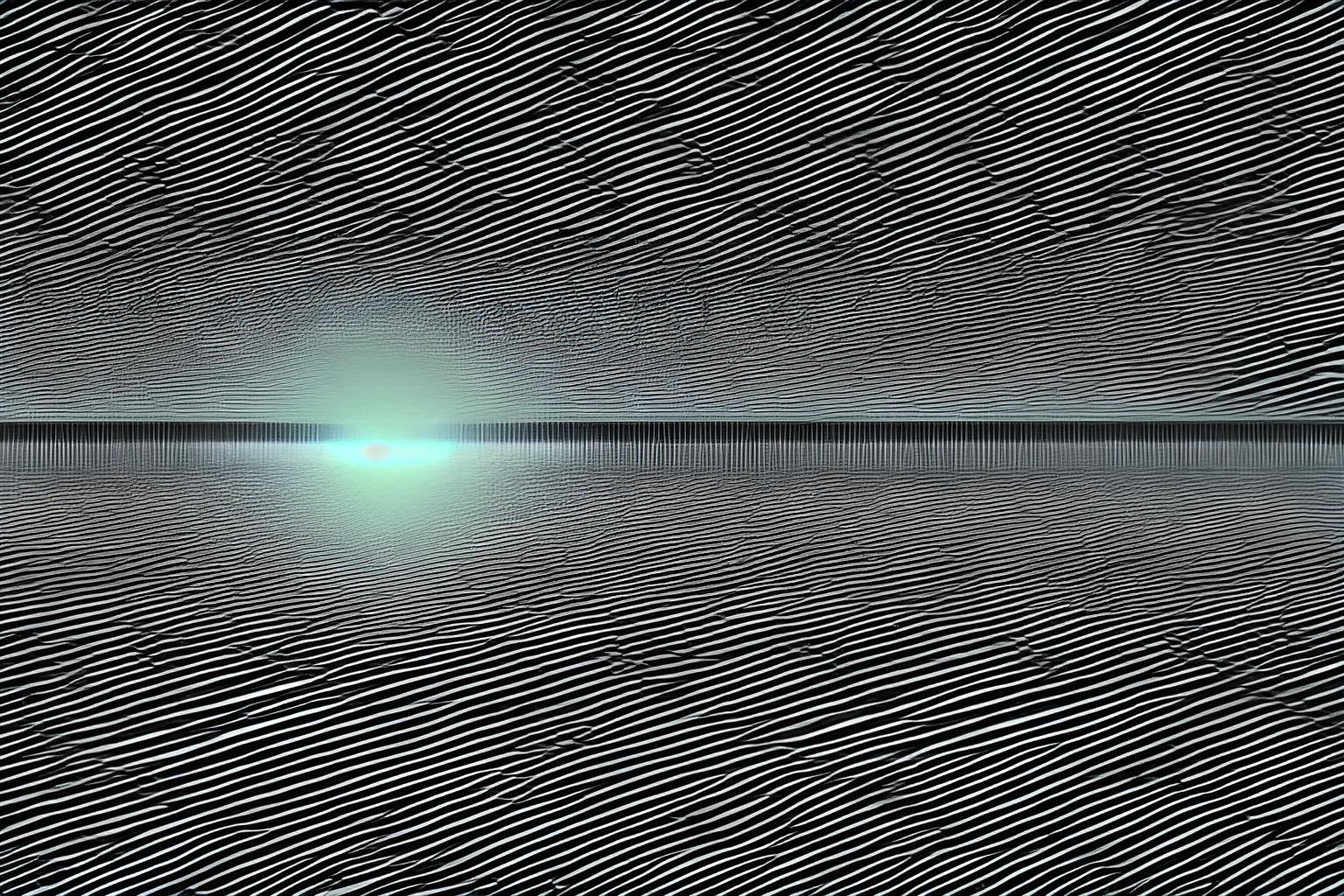
The Story of Earth
Chapter 1 - The Big Bang
Contents
The Big Bang is the theoretical starting point of our observable universe. It occurred about 13.8 billion years ago with the abrupt appearance of expanding spacetime.
The age of the universe is estimated using various methods, including the cosmic microwave background (CMB) radiation, the Hubble constant, the ages of the oldest stars, and the age of the oldest globular clusters. The most precise estimate comes from the CMB, which gives an age of about 13.8 billion years. The latest data from the James Webb Telescope indicate incredibly large galaxies were present in the early universe which contradicts what scientist expected to find in very young, hot, dense universe. The scientific implications of this discovery are complicated, finding elements like oxygen earlier than expected might mean early processes happened much faster than models predicted.
The Big Bang
1. The Start of the Universe
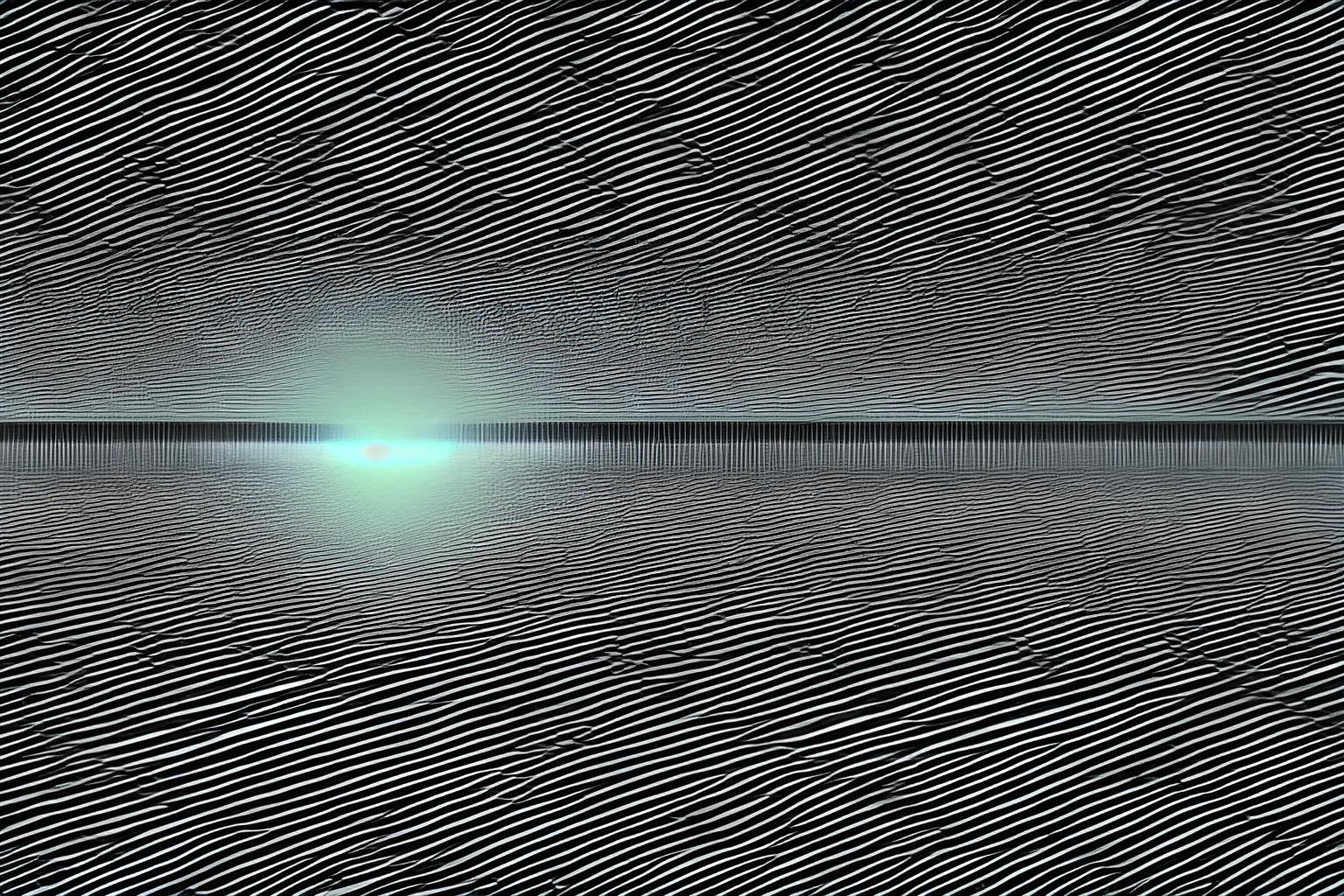
Stages of the Big Bang
Theorized Stages of Universe Evolution
The image above represents the theorized stages in the evolution of the universe. The infant universe was an extremely hot, dense, nearly homogeneous mixture of photons and matter, tightly coupled together as a plasma.
-
Inflationary era: The universe undergoes an extremely rapid period of expansion, smoothing out the density of matter and radiation.
It is believed to have lasted for a fraction of a second, during which the universe underwent a rapid and exponential expansion.
The idea of cosmic inflation was first proposed by physicist Alan Guth in 1980, in order to explain a number of problems with the standard Big Bang model. Specifically, the standard model could not explain the large-scale homogeneity and isotropy of the universe, or the flatness of space-time.
The evidence for cosmic inflation comes from a variety of observations, including the cosmic microwave background radiation, the large-scale structure of the universe, and the polarization of the cosmic microwave background. These observations provide strong support for the idea that the universe underwent a brief period of exponential expansion in its early history.
-
During this era, the universe was cool enough for protons and neutrons to combine and form atomic nuclei, but still hot enough that most of the universe was in the form of free protons and neutrons.
This era began when the temperature of the universe had cooled to around 10^9 Kelvin. At this temperature, the protons and neutrons in the universe had enough energy to overcome their electromagnetic repulsion and fuse together into atomic nuclei. The most abundant elements produced during this era were hydrogen and helium, which formed in a ratio of about 3:1.
During the era of nucleosynthesis, the universe was too hot and dense for stable atoms to form, but the production of atomic nuclei was an important step towards the eventual formation of atoms.
-
The Dark Ages is a period in the early universe, when there were no sources of light or radiation, and the universe was filled with neutral hydrogen gas. This period lasted from around 380,000 years after the Big Bang to around 150 million years after the Big Bang.
At the beginning of the Dark Ages, the universe had cooled enough that electrons and protons could combine to form neutral hydrogen atoms. This process, called recombination, released a burst of energy in the form of radiation, which eventually became the cosmic microwave background radiation.
During the Dark Ages, the universe continued to expand and cool, and small fluctuations in the density of matter began to grow through the force of gravity. These fluctuations eventually led to the formation of the first stars and galaxies, which began to emit radiation and heat, ending the era of darkness.
-
The Era of Reionization is a period in the history of the universe that occurred around 150 million to 1 billion years after the Big Bang. During this era, the first stars and galaxies began to form and emit intense radiation, which reionized the hydrogen gas in the universe.
At the beginning of the era of reionization, the universe had cooled enough that the first clumps of gas began to collapse under their own gravity, forming the first galaxies and stars. These objects emitted intense radiation, including ultraviolet light, which ionized the hydrogen gas in the surrounding universe.
The era of reionization was a crucial period in the history of the universe, as it marked the transition from a universe dominated by dark matter to a universe filled with visible matter in the form of stars and galaxies.
-
The era of the first stars and galaxies is a period in the history of the universe that occurred roughly 150 million to 1 billion years after the Big Bang.
The first clumps of gas began to form as small fluctuations in the density of matter grew through the force of gravity. These fluctuations eventually led to the formation of the first protogalaxies and the first stars.
The first stars to form were likely much more massive than the stars we see today, with masses up to 100 times that of the Sun. These massive stars burned through their nuclear fuel at a rapid pace and had short lifetimes, exploding as supernovae after only a few million years. The explosions of these first-generation stars released heavy elements, such as carbon and oxygen, into the surrounding gas, enriching it with metals that would form the building blocks of later generations of stars and planets.
-
The accelerated expansion phase of the Big Bang is a period in the history of the universe that began about 9 billion years after the Big Bang and is ongoing. During this phase, the expansion of the universe began to accelerate at an increasingly rapid rate.
The discovery of this acceleration came as a surprise to astronomers, who had expected the expansion of the universe to gradually slow down due to the gravitational attraction of matter. However, observations of distant supernovae and measurements of the cosmic microwave background radiation showed that the expansion of the universe was actually speeding up.
The cause of this acceleration is still not fully understood, but it is thought to be driven by a mysterious form of energy called "dark energy." Dark energy is a hypothetical form of energy that permeates all of space and has a negative pressure, causing it to drive the acceleration of the universe.
The accelerated expansion phase is an area of active research and study, with astronomers and physicists working to understand the nature of dark energy and its effects on the universe.
Dating the Big Bang
The Anisotropies of the Cosmic Microwave Background (CMB)
The CMB false color image above is a snapshot of the oldest light in our cosmos, imprinted on the sky when the Universe was just 380 000 years old. It shows tiny temperature fluctuations that correspond to regions of slightly different densities, representing the seeds of all future structure: the stars and galaxies of today.
-
The cosmic microwave background radiation (CMB) is a faint glow of light that permeates the entire universe, and is thought to be the leftover radiation from the Big Bang. The temperature of the CMB is nearly uniform in all directions, with small fluctuations that provide information about the early universe. The precise measurement of these fluctuations can be used to determine the age of the universe.
The current best estimate of the age of the universe from the CMB is about 13.8 billion years. This estimate is based on data from several experiments, including the Wilkinson Microwave Anisotropy Probe (WMAP) and the Planck satellite.
-
The Hubble constant is a measure of the rate at which the universe is expanding, and is based on observations of the redshift of distant galaxies. The redshift is a measure of how much the light from a galaxy has been stretched as the universe expands, and is directly proportional to the distance to the galaxy. By measuring the redshift and distance of many galaxies, astronomers can estimate the Hubble constant and use it to estimate the age of the universe.
The most recent measurements of the Hubble constant give an age of about 13.8 billion years, consistent with the age estimated from the CMB.
-
The ages of the oldest stars in the universe can be estimated by observing their brightness, temperature, and chemical composition. The oldest stars are thought to have formed shortly after the Big Bang, and their ages can provide a lower limit on the age of the universe.
The oldest known stars in the Milky Way are estimated to be about 13.5 billion years old, which gives a lower limit on the age of the universe.
-
Globular clusters are dense, spherical collections of stars that are thought to have formed early in the history of the universe. By measuring the ages of the oldest globular clusters in the Milky Way and other galaxies, astronomers can estimate the age of the universe.
The age of the oldest globular clusters is estimated to be about 12.7 billion years, which provides another lower limit on the age of the universe.
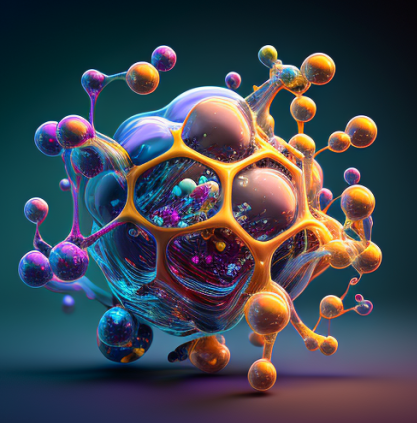
Fundamental particles, also known as elementary particles, are the building blocks of matter and energy. They are described by the Standard Model of particle physics, which is a theoretical framework that describes the behavior of these particles and their interactions with each other. Fundamental particles are classified into two categories: fermions which build matter and bosons which exchange energy like radiation and light. The particles have different properties because they have different values for spin, a property of quantum mechanics relating to angular momentum.
The Big Bang
2. Fundamental Particles
Fermions - Matter Makers
Fermions combine to form matter because their half-integer spin values (e.g. 1/2, 3/2, etc.) make them governed by the Pauli exclusion principle. It states no two fermions can occupy the same quantum state simultaneously. There are two types of fermions: Quarks and Leptons
-
Quarks are particles that come in six different types, or "flavors": up, down, charm, strange, top, and bottom. Quarks are never found in isolation in nature, but always exist in bound states, such as protons and neutrons, or in other exotic particles that can be created in high-energy particle accelerators.
The up and down quarks are the lightest and most common of the six quarks and are the building blocks of protons and neutrons, which make up the nucleus of an atom.
-
Leptons are particles that do not interact via the strong force and include electrons, muons, and tau particles, as well as their associated neutrinos. Leptons are fundamental particles and are not composed of smaller particles. Electrons, for example, are the basic building blocks of atoms and are responsible for the chemical properties of matter, while neutrinos are nearly massless particles that interact very weakly with matter and are produced in nuclear reactions in the sun and other stars.
Bosons - Force Carriers
Bosons mediate interactions between fundamental particles. Unlike Fermions, they have whole integer spin values (e.g. 1, 2, etc.) meaning they can occupy the same quantum state. They form a unique state of matter known as a Bose-Einstein condensate.
Bosons include the photon, which mediates the electromagnetic force, the W and Z bosons, which mediate the weak force, and the gluon, which mediates the strong force.
-
Photon: The photon is a massless particle that mediates the electromagnetic force. It has a spin of 1 and is responsible for light and other forms of electromagnetic radiation.
-
W and Z bosons: The W and Z bosons are massive particles that mediate the weak force, which is responsible for radioactive decay. They have spins of 1 and 0, respectively.
-
Gluon: The gluon is a massless particle that mediates the strong force, which binds quarks together to form protons and neutrons. It has a spin of 1 and is responsible for the confinement of quarks.
-
Higgs boson: The Higgs boson is a massive particle that was discovered in 2012. It is associated with the Higgs field, which gives particles mass, and is thought to be responsible for the mechanism by which particles acquire mass. It has a spin of 0.
*** Explore Particles in Virtual Reality ***
-
The Standard Model
The Standard Model is a theoretical framework in particle physics that describes the fundamental particles and their interactions through the strong, weak, and electromagnetic forces. It consists of three families of particles: quarks and leptons, which make up matter, and bosons, which mediate the fundamental forces.
-
What shape is a quark?
Quarks are elementary particles that are considered to be the building blocks of matter. They are not typically described as having a specific shape. Instead, they are often represented mathematically as point-like particles, meaning they have zero size and are treated as having no spatial extent.
-
How to illustrate spin?
It is known that quarks have a property called spin, which is a measure of their intrinsic angular momentum. This property is related to the way that quarks interact with other particles. Overall, the concept of shape is not typically used to describe quarks, but it can be useful to experiment with different visual styles.
-
Quarks combine to form Hadrons
Quarks combine to form composite particles called hadrons, which include protons, neutrons, and various other types of particles. The combination of quarks is governed by the strong nuclear force, which is one of the four fundamental forces of nature. This force binds the quarks together through the exchange of particles called gluons.
-
Protons
Protons are one of the three subatomic particles that make up atoms, along with neutrons and electrons. They have a positive electric charge and are slightly lighter than neutrons. Protons are essential components of atomic nuclei and determine the identity of an element. Protons are composed of two up quarks and one down quark, which gives them a total electric charge of +1. The strong nuclear force binds the quarks together to form a stable proton, along with the neutrons that make up the nucleus of an atom.
-
Neutrons
Neutrons are composed of three quarks: two down quarks and one up quark. The total electric charge of the three quarks in a neutron is therefore zero, giving the neutron its neutral charge. The strong nuclear force binds the quarks together to form a stable neutron, along with the protons that make up the nucleus of an atom. The study of the quark composition of neutrons and other particles is an important area of research in particle physics, as it helps to deepen our understanding of the fundamental building blocks of matter and their interactions.
The Big Bang
3. Anatomy of Atoms
As the universe continued to expand and cool, groups of three quarks clumped together to form protons and neutrons, the foundation of matter.
The nucleus is the central core of an atom, made up of protons and neutrons. It is the most dense and most massive part of the atom, occupying only a tiny fraction of the total volume of the atom. The number of protons in the nucleus determines what element the atom is, as each element has a unique number of protons in its nucleus.
Electrons are negatively charged subatomic particles that are found outside the nucleus of an atom in the electron cloud. The electrons in an atom are arranged in energy levels or shells, with each shell being a certain distance from the nucleus. The electrons in the innermost shell are the closest to the nucleus and have the lowest energy, while the electrons in the outermost shell are farthest from the nucleus and have the highest energy.
-
Protons are positively charged particles that are located in the nucleus, or center, of an atom. Each element has a specific number of protons in its nucleus, known as its atomic number, which determines the chemical properties of that element. The number of neutrons in the nucleus can vary, and atoms with the same number of protons but different numbers of neutrons are known as isotopes.
Protons form from combination of two up quarks and one down quark. Its mass is slightly less than a neutron but much higher than an electron. Protons are held together in the nucleus by the strong force carried by gluons
-
Neutrons are neutral particles that are also located in the nucleus of an atom. Together with protons, they make up the bulk of the mass of an atom. The number of neutrons in an atom can vary, resulting in isotopes of the same element that have different atomic masses. They form from a combination of two down quarks and one up quark. The mass is slightly larger than a proton. Neutrons play an important role in the stability of atomic nuclei.
-
Electrons are negatively charged particles that orbit the nucleus in shells or energy levels. Electrons are responsible for the chemical behavior of atoms, as they determine how atoms bond with one another to form molecules. The number of electrons in an atom is typically equal to the number of protons, which gives the atom a net charge of zero. Electrons are fundamental particles. Their mass is 1/1836 of a proton. Electrons in the outer shell can be shared to form bonds with other atoms.
The Big Bang
4. The First Six Elements
As the universe continued to expand and cool, matter began to coalesce into stars. The first stars formed around 200-400 million years after the Big Bang, and over time, they synthesized heavier elements and eventually exploded as supernovas, releasing these elements back into space. The first six elements H, He, Li, C, O, and N formed from the first phase of star growth.
-
The first and is the most basic element, Hydrogen, has one proton within the nucleus. The number of neutrons can vary, these variations are called isotopes. (See Chapter 3 for the Fundamentals of Isotopes). The single proton of hydrogen gives it a positive charge of +1.
-
The second element formed when two hydrogen atoms collided but instead of breaking apart, two protons fused into one nucleus creating a positive charge of +2. The way electrons couple with positive nuclei to form atoms is different for each element. The distribution of electrons around the nucleus is called the electron configuration and determines how atoms bond.
-
The third element, Lithium, produced in trace quantities in the early universe, contains 3 protons.
Lithium, is the the first of the Alkali Metals Group on the far left of the periodic table, all of which tend to lose one outer electron resulting in the formation of a positive ion. At room temper they are shiny and so soft they can be easily cut with a knife. They are stored under oil because they are so highly reactive. Freshly cut surfaces tarnish rapidly due to interaction with oxygen in the air. They are never found as native elements, only bonded to other elements to form salts.
-
The next element jumps ahead to Carbon with 6 protons. Because of the distribution of electrons carbon can have it can bond in several different ways. We will look closer at the bonding styles of carbon when the first minerals are formed.
*Carbon formation in the universe is thought to have occurred primarily through the process of stellar nucleosynthesis, which takes place in the cores of stars, late in a star’s life, when the core temperature and pressure become extremely high, carbon can be synthesized from helium through a series of fusion reactions. It is believed to have started several billion years after the Big Bang. This is because the first generation of stars consisted mostly of hydrogen and helium, and it took time for heavier elements to be produced through stellar nucleosynthesis and then distributed throughout the universe through processes such as supernova explosions
-
The fifth element formed during the early universe was Oxygen with 8 protons
Along with carbon, oxygen formed as a byproduct of the collapse of the first stars. The nucleus of oxygen can be made by fusing four helium cores together.
-
The sixth element and last in the first phase of element formation is Nitrogen. It formed from the collapse of stars containing carbon and oxygen. Nitrogen contains seven protons.
A molecule is a group of two or more atoms that are bonded together through shared electrons. The atoms can be of the same element, as in the case of oxygen molecules (O2) or nitrogen molecules (N2), or they can be of different elements, such as water (H2O) or carbon dioxide (CO2). The bond type and strength is influenced by several factors including the position of the shared electrons with respect to the atoms, the electronegativity of the atoms, and their atomic radii.
The difference of electronegativity between bonded electrons determines the bond type for non-metallic bonds. Bonds between the same atom, or atoms that have similar electronegativities form Non-Polar Covalent Bonds, if the difference is greater than 0.5 but less than 1.8 the bond will be Polar Covalent. Bonds between atoms with a difference of electronegativities greater than 1.8 result in Ionic Bonds. They are strongly polar.
The Big Bang
5. Elements Bond Together in New Ways
-
Nonpolar covalent bonds are characterized by a symmetrical distribution of electrons between the two atoms. This means that the electrons spend an equal amount of time near each atom, creating a balanced distribution of charge. As a result, nonpolar covalent compounds are often electrically neutral and do not have a dipole moment.
The properties of nonpolar covalent compounds can be influenced by the size and shape of the molecules. For example, nonpolar molecules tend to be hydrophobic and do not dissolve easily in water, as they are not able to form hydrogen bonds with the water molecules. Nonpolar molecules also tend to have lower melting and boiling points than polar compounds, as they do not have the same degree of intermolecular attractions.
Examples of nonpolar covalent compounds include diatomic molecules (molecules made of two atoms of the same element) such as H2, O2, and N2, as well as hydrocarbons such as methane (CH4) and ethane (C2H6). In these compounds, the atoms are held together by covalent bonds in which the electrons are shared equally between the atoms.
-
Polar covalent bonding is a type of chemical bonding in which electrons are shared unequally between two atoms. The atoms involved have different electronegativities, which means one atom has a greater tendency to attract electrons towards itself than the other atom. As a result, there is a separation of charge between the atoms, and the bond is said to be polar.
Polar covalent bonds are characterized by an asymmetrical distribution of electrons between the two atoms. This means that the electrons spend more time near the atom with the higher electronegativity, creating a partial positive charge on one atom and a partial negative charge on the other. This results in a dipole moment, or a separation of charge, across the molecule.
Examples of polar covalent compounds include water (H2O), ammonia (NH3), and hydrogen chloride (HCl).
The properties of polar covalent compounds can be influenced by the degree of polarity in the molecule. For example, polar molecules tend to be hydrophilic and dissolve easily in water, as they are able to form hydrogen bonds with the water molecules. Polar molecules also tend to have higher melting and boiling points than nonpolar compounds, as they have stronger intermolecular attractions.
-
Ionic bonding is a type of chemical bonding that occurs between a metal and a nonmetal where one atom loses one or more electrons to another atom, resulting in the formation of positively charged cations and negatively charged anions held together by strong electrostatic forces of attraction, forming an ionic compound.
The process of ionic bonding involves the transfer of electrons from the valence shell of one atom to the valence shell of another atom. This typically occurs when the electronegativity difference between the two atoms is large.
Ionic compounds tend to have high melting and boiling points, due to the strong electrostatic forces between the ions. They are also typically brittle, as the ionic lattice can easily shatter when subjected to stress. In the solid state, ionic compounds do not conduct electricity, as the ions are held in a fixed position and cannot move freely. However, when dissolved in water or in the molten state, ionic compounds can conduct electricity, as the ions are free to move and carry electrical charge.
Examples of common ionic compounds include sodium chloride (NaCl), calcium carbonate (CaCO3), and magnesium oxide (MgO). In these compounds, the metal atom (sodium, calcium, or magnesium) donates one or more electrons to the nonmetal atom (chlorine, carbon, or oxygen), resulting in the formation of cations and anions that are held together by ionic bonds.
-
Metallic bonding is a type of chemical bonding that occurs between metal atoms. In metallic bonding, the valence electrons of the metal atoms are delocalized and are free to move throughout the solid metal lattice. This results in a network of positively charged metal ions surrounded by a sea of negatively charged delocalized electrons.
The strength of metallic bonding arises from the attraction between the positively charged metal ions and the negatively charged electrons that surround them. The delocalized electrons are not bound to any one metal ion, but instead move freely throughout the lattice, creating a pool of electrons that are shared by all the metal ions. This results in a highly coordinated and strong bond between the metal ions.
The delocalized electrons in a metallic bond have several important properties that contribute to the unique characteristics of metals. For example, they are mobile and can move throughout the lattice in response to an applied electric field, which makes metals good conductors of electricity. They are also responsible for the high thermal conductivity of metals, as they can transfer energy throughout the lattice by colliding with neighboring ions.
In addition to their unique physical properties, metals also exhibit a range of other important behaviors due to their metallic bonding. For example, metals are typically malleable and ductile, meaning that they can be easily shaped and deformed without breaking. This is because the metallic bonding is able to deform and adjust to changes in shape without breaking the lattice structure. Metals are also typically dense and have high melting and boiling points, due to the strong metallic bonds between the atoms.
Overall, metallic bonding is an important type of chemical bonding that plays a critical role in the structure and properties of metals. The properties of metallic compounds can be explained by the delocalized electrons that contribute to the strength of the metallic bond and allow metals to exhibit unique properties such as electrical conductivity, thermal conductivity, malleability, and ductility.
-
Single bond: A covalent bond in which two atoms share one pair of electrons. It is the simplest type of covalent bond and is often found in small molecules and organic compounds.
-
Double bond: A covalent bond in which two atoms share two pairs of electrons. It is relatively stronger and longer than a single bond and is commonly found in larger molecules and compounds, such as those containing carbon-carbon double bonds or carbon-oxygen double bonds.
-
Triple bond: A covalent bond in which two atoms share three pairs of electrons. It is even stronger and shorter than a double bond and is often found in molecules such as nitrogen gas (N2) and acetylene (C2H2).
-
Coordinate covalent bond: A covalent bond in which one atom donates both electrons to be shared with another atom. This type of bond is also known as a dative bond and is often found in molecules containing metal ions or other positively charged species.
-
Pi bond: A covalent bond that forms when two atomic orbitals overlap sideways to share electrons above and below the plane of the atoms. This type of bond is weaker than a sigma bond and is often found in double and triple bonds.
-
A sigma bond is a type of covalent bond that forms when two atomic orbitals overlap end-to-end to share electrons along the axis between the two atoms. In a sigma bond, the electrons are shared symmetrically between the two atoms, and the bond is often represented as a single line between the two atoms.
Building Elements and Molecules in VR
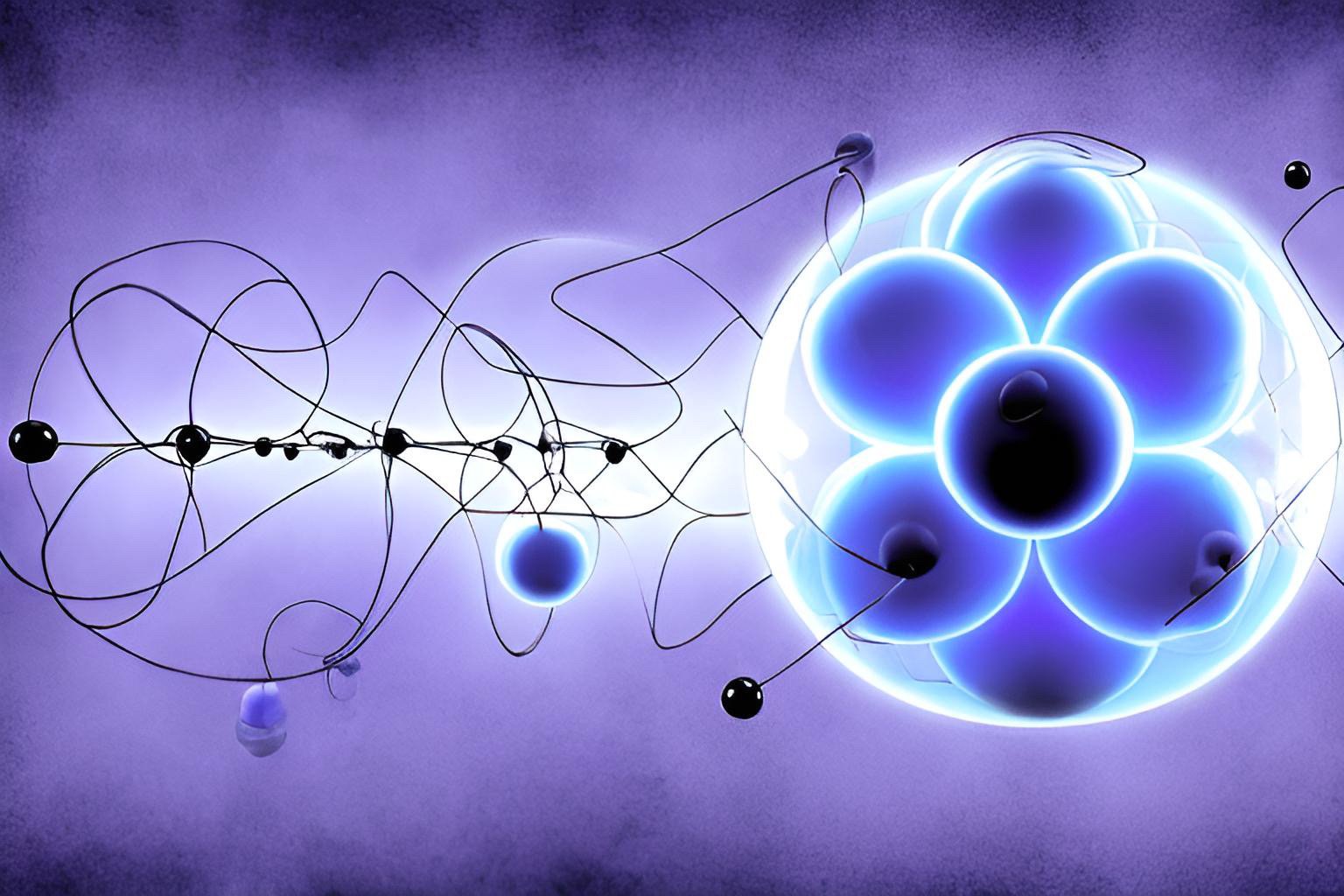
The First Molecules
The first elements bonded together to form the first Molecules. They became more and more complex as new elements formed and spread throughout the universe through star formation. Below are a few examples of the first compounds formed from the first elements in the universe. Some of these molecules are still abundant on earth today, others only exist in extreme high temperature/density regions of space.
-
HeH+ diatomic molecule consisting of a helium atom and a hydrogen ion. It is the first molecular ion to have been formed in the universe, shortly after the Big Bang and it is believed to have played a key role in the formation of the first stars and galaxies. HeH+ was first observed in the laboratory in 1925 by the German physicist Walter Heitler and the Danish physicist Fritz London, who used quantum mechanics to predict its existence. The interaction between helium and hydrogen ion is very complex, involving both Coulombic and quantum effects. The molecule has a dipole moment, which means that it has a positive and a negative end, and is therefore polar.
H2+ is a molecular ion in which one of the hydrogen atoms has lost an electron, resulting in a positive charge. As a result, the two hydrogen atoms are held together by an electrostatic attraction between the positively charged nucleus of the ion and the negatively charged electron of the other hydrogen atom. This results in a polar molecule, with a positive charge at one end and a negative charge at the other end. Due to its electronic structure, H2+ is a highly reactive molecule, and is prone to undergoing chemical reactions with other molecules or ions.
H2 is a neutral molecule in which the two hydrogen atoms share electrons to form a covalent bond. The electrons are distributed between the two atoms in such a way that the molecule has a stable, nonpolar configuration. H2 is relatively stable, and is typically inert under normal conditions.
H3+ is also known as protonated molecular hydrogen or the triatomic hydrogen ion because it consists of three hydrogen atoms, one of which is ionized and has lost an electron, resulting in a positive charge. The positive charge is often referred to as a proton, hence the name "protonated". The ionized hydrogen atom acts as a proton making it a highly reactive molecule that can interact with other ions or neutral molecules. It can act as an acid in certain reactions, donating a proton (H+) to a base molecule. It is also involved in various organic reactions, such as proton transfer reactions and carbocation rearrangements. H3+ is the most abundant molecular ion in the universe. It is formed in interstellar space by a series of ion-molecule reactions, and can be detected by astronomers using spectroscopic techniques. The abundance and distribution of H3+ in space provides insights into the physical and chemical conditions of the interstellar medium, and can help us understand the processes that lead to the formation of stars and planets.
LiH (lithium hydride) is an inorganic compound that consists of a lithium atom and a hydrogen atom. It is a white, crystalline solid that is highly reactive and can react violently with water or air. It is highly polar creating a strong electric dipole moment. This makes LiH an important source of hydrogen in fuel cells and as a moderator in nuclear reactors. It also has applications in the field of solid-state physics, where it is used as a model system for studying the behavior of other ionic solids.
-
CH+ (methylidynium ion) forms through a reaction between hydrogen (H) and carbon (C) atoms. Today, CH+ is still found in a variety of environments, including interstellar space, planetary atmospheres, and comets.
CH is a diatomic molecule composed of a carbon atom and a hydrogen atom. The CH radical plays an important role in atmospheric chemistry, where it is involved in the formation of ozone and other pollutants. It is also of interest to astrochemists studying the chemistry of the interstellar medium, where it is an important intermediate in the formation of more complex organic molecules.
CN is composed of one carbon atom and one nitrogen atom. , and is also a diatomic molecule. It is a relatively stable molecule that is found in the Earth's atmosphere, in interstellar space, and in the tails of comets. It has a triple bond between the carbon and nitrogen atoms, which makes it a stronger and more stable molecule than CH.
C2 is a linear molecule composed of two carbon atoms, which are triple-bonded together with a total of two pi bonds. It is commonly referred to as ethynyl or acetylene, and is a highly reactive molecule that is used in a variety of industrial processes, such as welding and cutting.
C3 is composed of three carbon atoms, which can exist in several different forms. The most common isomer is propynyl or methylacetylene, which has a linear structure similar to that of C2 but with an additional carbon atom. Another isomer is cyclopropenylidene, which has a cyclic structure with all three carbon atoms in a ring. C3 is a more stable molecule than C2 due to the presence of additional carbon-carbon bonds, and is also involved in various chemical reactions, such as in the formation of larger organic molecules in the interstellar medium.
CH2+ (methylenium ion) is a highly reactive, positively charged molecular ion that consists of a carbon atom and two hydrogen atoms. It can form in a variety of ways, including the dissociation of larger organic molecules, the reaction of a neutral carbon atom with a molecule such as methane or hydrogen, and the reaction of a proton with a molecule such as ethylene. Once formed it is an important intermediate in many chemical reactions such as the formation of polycyclic aromatic hydrocarbons (PAHs) which is of interest to astrochemists studying the chemistry of the interstellar medium.
CH4 (methane) is a molecule composed of one carbon atom and four hydrogen atoms, with a tetrahedral structure. It is the simplest hydrocarbon and is the primary component of natural gas.Description text goes here
-
-OH (hydroxyl) is a molecule consisting of one oxygen atom and one hydrogen atom. It is a highly reactive and unstable species involved in many chemical and biological processes. The hydroxyl group (-OH) is a common functional group in organic chemistry, and is present in many organic compounds such as alcohols and carboxylic acids. It is also a key component in many biochemical molecules, such as amino acids, nucleic acids, and carbohydrates.
H2O (water)
CO is a molecule composed of one carbon atom and one oxygen atom, with a linear structure. It has been detected in various astronomical environments, including comets, planetary atmospheres, and interstellar clouds. It is thought to play a role in the formation of larger organic molecules in space, and has been proposed as a possible biomarker for detecting life on other planets.
CO2 (carbon dioxide) is a linear molecule with a carbon atom at the center and two oxygen atoms arranged in a straight line on either side of it. The carbon-oxygen bonds in CO2 are covalent, meaning that the atoms share electrons in order to form the bonds. It is relatively unreactive under normal conditions, but it has the ability to absorb and emit infrared radiation making it a large influence on atmospheric conditions.
H2CO (formaldehyde) is a colorless gas with a pungent odor. It consists of one carbon atom, two hydrogen atoms, and one oxygen atom.
HCOOH (formic acid) is a colorless liquid with a pungent odor. Its molecular formula is HCOOH, meaning it consists of one carbon atom, one hydrogen atom, and two oxygen atoms.
-
N2 nitrogen gas, is a colorless and odorless diatomic gas that makes up about 78% of the Earth's atmosphere. It consists of two nitrogen atoms held together by a triple covalent bond, which is one of the strongest chemical bonds found in nature. This makes N2 a relatively unreactive molecule under normal conditions.
NH3 (Ammonia) - A colorless and pungent gas with the chemical formula NH3, ammonia is a compound of nitrogen and hydrogen, and it is one of the most widely produced and important industrial chemicals in the world.