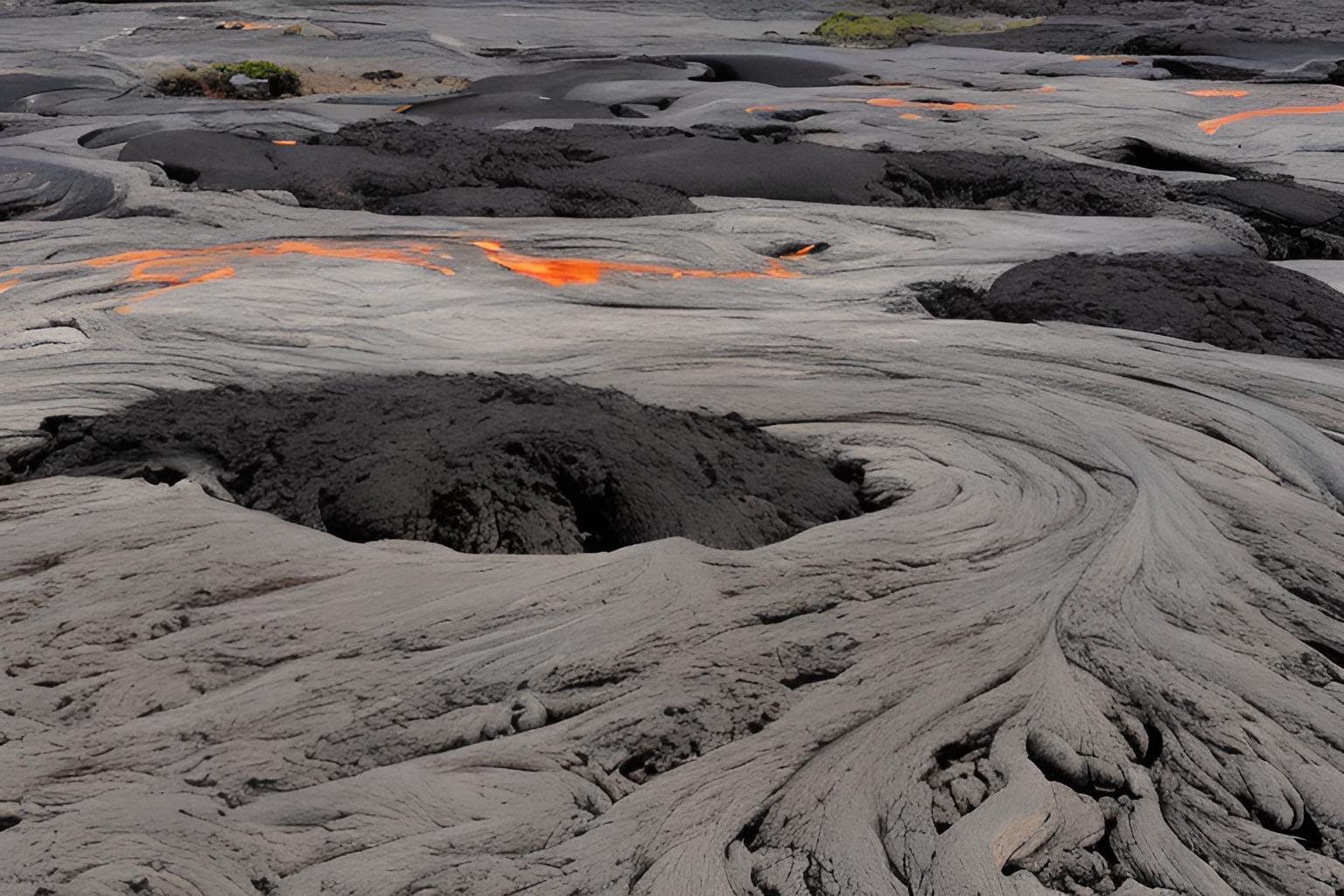
Chapter 4. proto-Earth
1.proto-Earth
Proto-Earth refers to the early stage of Earth's formation, before it reached its current size and composition. It was a time when our planet was still in the process of forming through the accumulation and collision of various celestial objects like meteorites, comets, asteroids, and planetesimals. We use the term "proto-Earth" to describe the planet around 4.6-4.5 billion years ago during its initial stages of development up to the collision with Theia (Chapter 5). It marks the beginning of the Hadean Eon. The name "Hadean" is derived from "Hades," the ancient Greek god of the underworld, reflecting the harsh and inhospitable conditions thought to have existed on Earth during this time.
-
-
As we saw in in Chapter 3, the formation of proto-Earth began with the process of accretion, in which dust and gas from the protoplanetary disk surrounding the young Sun collided and stuck together to form larger bodies called planetesimals. Over time, these planetesimals continued to collide and merge, eventually forming the early proto-Earth, along with other protoplanets in our solar system.
-
The proto-Earth's composition was influenced by the materials it accumulated during the accretion process, which included silicate minerals, metals, water, volatile elements, and possibly organic compounds. These materials came from various celestial objects like meteorites, comets, and planetesimals. We will cover each material in more detail throughout the chapter.
-
As the proto-Earth grew in size, it experienced an increase in heat generated by the energy from collisions and radioactive decay of isotopes within the planet. This heating led to the differentiation of the Earth's interior into layers of different densities. The denser materials, like iron and nickel, sank to form the core, while lighter silicate minerals rose to form the mantle and eventually the crust. We will explore the fundamentals of the core in Chapter 7.
-
The proto-Earth's atmosphere was initially composed of light elements like hydrogen and helium, as well as volatile elements like water vapor, carbon dioxide, and methane. We will explore the topic of water on Earth in Chapter 6.
Over time, the outgassing from the planet's interior through volcanic eruptions changed the composition of the atmosphere and hydrosphere.
-
The most significant event in the history of proto-Earth was the likely impact between a Mars-sized body called Theia around 4.5 billion years ago. This catastrophic collision resulted in the formation of debris that eventually coalesced to form the Moon. We will cover the fundamentals of the moon in the next chapter, Chapter 5.
2.Composition
The early Earth's composition was dominated by the materials found in the protoplanetary disk, such as meteorites, comets, and other protoplanets, as discussed in Chapter 3. We can use the composition of meteorites found on earth dating to the beginning of the solar system 4.6 billion years ago as a starting point for Earth’s composition. Determining the exact composition of the early Earth is challenging, because no rock samples and only a few grains of crystals have been preserved on the surface from this time.
-
The Hadean Eon is characterized by a lack of preserved rock formations, which is one of the reasons why studying this period of Earth's history is so challenging. Most of the rocks that formed during this time have since been destroyed by geological processes such as erosion, subduction, and metamorphism. However, a few ancient zircon crystals have been discovered and dated to be around 4.4 billion years old, providing some clues about the conditions of the Earth during this time. We will discuss the significance of these crystals in Chapter 6.
During the Hadean Eon, the Earth's mineral composition was different from today, but many of the main rock forming mineral groups were present. Silicate minerals like quartz, olivine, and feldspars, were abundant. We will discuss these mineral groups in detail later this chapter.
Other mineral groups were likely present including sulfide minerals formed due to volcanic and hydrothermal activity. We will discuss this process in Chapter 8. Iron rich oxide minerals such as magnetite and hematite were likely present. Carbonate minerals like calcite and dolomite precepted from hot springs fed from deep in the Earth, which also accumulate native elements, including gold, platinum, and copper.
-
Water was likely delivered to the early Earth through the accretion of water-rich celestial bodies like comets and water-bearing asteroids. This water would have eventually contributed to the formation of Earth's oceans and played a crucial role in the development of its hydrosphere.
-
Iron and nickel, along with trace amounts of other metals, were also present in the early Earth's composition. These metals are thought to have come primarily from meteorites and other celestial bodies. As the Earth differentiated, the heavier elements, like iron and nickel, migrated to the core, while the lighter silicate minerals rose to form the mantle and crust. We will explore this process and the effects like magnetism in Chapter 7.
-
The early Earth also contained volatile elements, such as hydrogen, helium, carbon, nitrogen, and noble gases. These elements were either inherited from the solar nebula or delivered through comets and volatile-rich meteorites. They contributed to the formation of Earth's atmosphere and were essential for the development of life on the planet.
-
Organic compounds, including simple organic molecules like amino acids and hydrocarbons, have been found in meteorites and comets. These compounds may have been delivered to the early Earth and played a role in the origins of life. We will go over the earliest signs of life on earth when we explore the Paleozoic “Old Life” at the beginning of Part 2 of the Story of Earth.
Silicates are the cornerstone of Earth's geology, shaping our planet's crust and defining its unique composition, while driving the processes that have sculpted its dynamic landscapes over billions of years.
3.Sillicates
Silicates are a class of minerals that are composed primarily of silicon and oxygen, with the basic chemical formula of SiO4. These minerals make up the majority of Earth's crust, and are the most abundant type of mineral found on the planet. Silicates can be divided into various groups based on their structure. Before we explore the tetrahedral structure of SiO4 let’s go over some silicon based vocabulary:
-
Silicon is a chemical element with the symbol Si and atomic number 14. It is a metalloid, which means it has properties between those of metals and nonmetals. Silicon is the second most abundant element in Earth's crust, after oxygen. It is commonly found in the form of silicon dioxide (silica) and silicate minerals. Silicon is widely used in the electronics industry for semiconductors, as well as in the production of solar panels, glass, and various alloys.
-
Silica, also known as silicon dioxide (SiO2), is a chemical compound consisting of one silicon atom and two oxygen atoms. Silica occurs in both crystalline and amorphous forms, with quartz being a well-known example of the crystalline form. Silica is the primary component of sand and is used as a raw material in the production of glass, ceramics, and concrete. In nature, silica is commonly found in rocks, soils, and as a constituent of the Earth's crust.
-
Silicates are a group of minerals composed of silicon, oxygen, and one or more additional elements, such as aluminum, magnesium, iron, calcium, sodium, and potassium. The basic structural unit of silicate minerals is the silica tetrahedron (SiO4), in which a silicon atom is surrounded by four oxygen atoms. Silicate minerals can be classified into various groups based on the arrangement of these tetrahedra, such as nesosilicates, sorosilicates, cyclosilicates, inosilicates, phyllosilicates, and tectosilicates. Silicates make up the majority of Earth's crust and mantle and are crucial components of many rock types, such as granite, basalt, and gneiss.
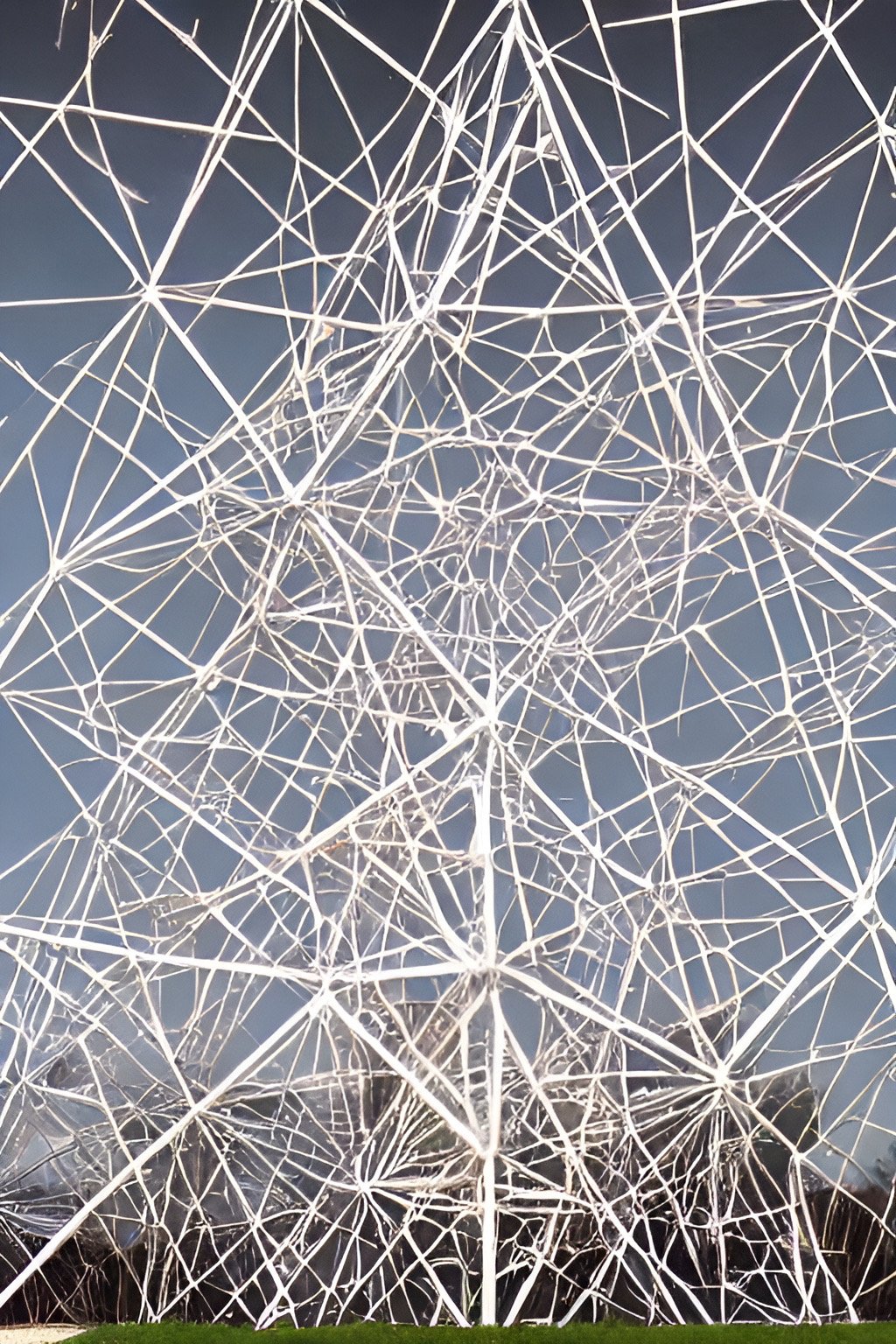
The Si-O Bond:
The electronegativity difference between oxygen and silicon in a Si-O bond within a silicate tetrahedron is 1.54, as calculated using the Pauling electronegativity scale. This value indicates that the Si-O bond has a polar covalent character, meaning that the electrons are shared unequally between the two atoms. In this bond, the oxygen atom attracts the electrons more strongly than the silicon atom, leading to an uneven distribution of electron density and the resulting polarity.
4.Tetrahedrals
The silicate tetrahedron consists of one silicon atom (Si) at the center and four oxygen atoms (O) surrounding it at the corners of a tetrahedron. The silicon atom forms covalent bonds with the oxygen atoms, resulting in the SiO4 unit with a net charge of -4.
This SiO4 tetrahedron is the basic building block of silicate minerals, which are the most abundant group of minerals in the Earth's crust. The tetrahedra can combine in various ways to form a wide range of silicate minerals with different structures and properties.
-
Nesosilicates (isolated tetrahedra): The tetrahedra do not share any oxygen atoms with each other and are bonded to other cations (such as magnesium or iron) to balance the charge. Examples include olivine and garnet.
-
Sorosilicates (double tetrahedra): Two silicate tetrahedra share one oxygen atom, creating an Si2O7 unit with a net charge of -6. An example is the mineral epidote.
-
Inosilicates (single and double chains): The tetrahedra can link together to form single or double chains by sharing two oxygen atoms, respectively. Examples include pyroxene and diopside.
-
Ring silicates, also known as cyclosilicates, are a subclass of silicate minerals characterized by silicate tetrahedra (SiO4) that are arranged in rings. In these structures, the SiO4 tetrahedra share two oxygen atoms with neighboring tetrahedra, forming closed ring-like structures. The rings can have different sizes, with the most common ones consisting of 3, 4, or 6 tetrahedra. These rings are connected to other cations or complex groups, which provide charge balance and help define the overall structure of the mineral.
-
Double-chain silicates link together by two or three tetrahedra sharing oxygens. Examples include amphibole and tremolite.
-
Phyllosilicates (sheet silicates): The tetrahedra share three oxygen atoms and form continuous two-dimensional sheets. Examples include micas and clay minerals.
-
Tectosilicates (framework silicates): All four oxygen atoms in the tetrahedra are shared with neighboring tetrahedra, creating a three-dimensional framework structure. Examples include quartz and feldspars.
Shared Oxygens
Tetrahedral Structures
?.The Feldspar Family
-
Feldspars are a group of rock-forming minerals that constitute more than 60% of the Earth's crust, making them the most abundant mineral group on our planet. They are alumino-silicate minerals, composed of aluminum, silicon, and oxygen atoms, along with various metal ions such as potassium (K), sodium (Na), and calcium (Ca).
Feldspars can be divided into two main subgroups: alkali feldspars and plagioclase feldspars.
-
Alkali feldspars primarily contain potassium and sodium ions. The main end-members of this group are orthoclase (KAlSi3O8), which contains potassium, and albite (NaAlSi3O8), which contains sodium. Alkali feldspars exhibit a range of colors, from white to pink, depending on their composition and the presence of trace elements. They are commonly found in igneous rocks like granite and syenite, as well as in metamorphic rocks like gneiss and schist.
-
Plagioclase feldspars form a continuous series of compositions between albite (NaAlSi3O8) and anorthite (CaAl2Si2O8). They are characterized by the substitution of sodium and calcium ions within the crystal lattice. Plagioclase feldspars are common constituents of various rock types, including igneous rocks like basalt, andesite, and diorite, as well as metamorphic rocks like gneiss and schist.
DATING
Relative
By the 1830s geologists understood the Earth was old, very very old, but they had no method to determine exactly how old the Earth was.
Sediment Accumulation - 100 million years. One early method was to add up the thickness of the thickest possible section of each geologic period and estimate how long it would have taken for those sediments to accumulate giving some average rate. One of the many problems with this method is that there are vast periods of time not accounted for in the sediment record either due to non deposition or due to erosion of previously deposited material. These gaps in sedimentation are called unconformities. This method also couldn’t account for the vast period of time, before rock cooled enough and then eroded to form sediments.
Ocean salinity buildup - 80-100 million years. Irish physicist John Joly tried to calculate the age of the earth assuming the oceans began as freshwater and became saltier over time due to runoff from the land. This method doesn’t take into account the massive amount of salt removed from the world’s oceans through the formation of evaporates. These are salty formations that form as a result of evaporation of seawater leaving behind salt and other minerals which are then burred by additional sediments.
Thermodynamics - 20 million years. A famously incorrect estimate came from Lord Kelvin (the one who pioneered the concept of absolute zero and the Kelvin temperature scale). He assumed the earth started as a molten ball the same temperature as the sun and cooled at the rate of heat escaping the earth coming up to the surface. Early minors recognized the temperature of the Earth got hotter as they dug deep mines. The failure in his estimate came from assuming all the heat was original to the formation of the Earth. He did not account for the amount of radioactive heat generated within the mantle and within organic and radioactive sedimentary beds across the world. It took many years, but eventually thanks to the work of Henri Becquerel, Marie and Pierre Curie, along with Ernest Rutherford it was discovered that heat from radioactive decay was a major contributor to the total heat of Earth and today it is the only remaining source of heat, original heat from Earth’s formation dissipated billions of years ago.
Absolute - Radiometric Dating
Geologists Bertram Boltwood and Arthur Holmes determined radioactivity could provide a solution to the puzzle of the actual age of the Earth. Only a handful of naturally occurring elements spontaneously decay at rates slow enough to use in geologic dating. Physicists at the time, including Lise Meitner, were able to precisely determine the half life and decay pattern of these elements. By measuring the ratio between parent and daughter material and knowing the precise half-life of the material an accurate date can be determined. Around 1900 Boltwood’s experiments resulted in ages of between 400 Million years and 2.2 Billion Years confirming that the Earth was very very old. The best source of high quality radioactive material comes from igneous rocks, specifically zircon minerals within igneous rocks.
Uranium-Lead from zircon crystals (ZrSiO4): Dates from one million to 4.5 Billion years ago
Uranium238 transitions to Lead206 has a half-life of 4.47 billion years
Uranium235 transitions to Lead207 has a half-life of 710 million years
can compare the Lead206 Lead207 ratios
Potassium-Argon: as old as 1.2 million years ago. The decay of Potassium40 to Argon40
Rubidium-Strontium
Carbon14: Dates less than 50,000 years ago. Nitrogen14 transitions to Carbon14 when a rogue neutron from cosmic rays hits a nitrogen atom in the atmosphere, it can kick out a proton resulting in Carbon14 (with two extra neutrons). When a plant or animal is alive, the amount of Carbon14 within it’s system is equal to the amount in the atmosphere. When that organism dies, it stops taking in new Carbon14 and the carbon within the organism degrades to Carbon12. The ratio of Carbon14 to Carbon12 indicates how long that organism has been dead.
Errors - all radiometric dates come with error bars that represent how reproducible each result is +/- a certain number of years. This range represents the likely actual age of the sample.
Fundamentals of isotopes